Introduction
Muscle relaxants are routinely used to improve intubating conditions,1 to decrease the risk of patients’ laryngeal trauma,2 and to improve surgical conditions.3-6 Incomplete recovery from paralysis at the time of extubation, however, is a risk and is still widely ignored by many anesthetists. An obstruction of the upper airway7,8 and a reduced hypoxic ventilatory response9 are the leading clinical symptoms. Clinical studies show that a residual neuromuscular block at the end of anesthesia increases the incidence of critical respiratory events in the post anesthesia care unit.10-12 Observational trials13 as well as database analyses14-16 reveal an association between management of neuromuscular function and the risk of postoperative pulmonary complications, coma, and mortality. This manuscript covers the importance of neuromuscular blocking agents in the concept of balanced anesthesia, incidence and complications of post-operative residual paralysis reversal agents and appropriate dosing, and techniques of neuromuscular monitoring. Finally, a clinical algorithm will be introduced, based on the assumption that any concept to avoid residual paralysis must work within the time frame in which emergence from anesthesia normally can be managed, i.e., 10 min. The algorithm, suitable for every non-depolarizing neuromuscular blocking agent, is characterized by using quantitative neuromuscular monitoring. We also describe strategies for implementation of quality improvement programs for better management of neuromuscular transmission, which we believe could help improve postoperative respiratory outcomes.
Neuromuscular monitoring
The basic principle for preventing residual neuromuscular block is quantitative neuromuscular monitoring. The nerve is transcutaneously stimulated with the train-of four (TOF) or post-tetanic count (PTC) pattern depending on the depth of the neuromuscular block. Clinically, stimulation of the ulnar nerve and measurement of the elicited muscle contraction of the thenar via the thumb is mostly performed. TOF stimulation allows counting of the number of elicited muscle contractions (Train-of-count = TOFC) and the calculation of the ratio of the fourth to the first twitch response (TOFR).
The basic principle for preventing residual neuromuscular block is quantitative neuromuscular monitoring.
Visual or tactile assessment of the twitch responses is encompassed as qualitative neuromuscular monitoring. The result is therefore subjective and dependent on the anesthetist. A residual neuromuscular block of TOFR > 0.4 cannot be reliably detected even by an experienced anesthetist.17 Quantitative assessment of the train-of-four fade by neuromuscular transmission monitoring is the only suitable method to identify low but clinically meaningful levels of residual neuromuscular block.10 Mechanical techniques such as mechanomyography, acceleromyography (AMG), and kinemyography (KMG) are measuring twitch responses related to the muscle contraction, such as force, acceleration, or velocity. Electromyography (EMG) is measuring the compound muscle action potential. All techniques must be calibrated to increase the reliability of the measurement. Calibration includes identification of the optimal supramaximal stimulation current and ensures optimal signal to noise ratio.18 Historically, mechanomyography was the gold standard for measuring neuromuscular function. It is however accident-sensitive and therefore not feasible for everyday intraoperative use. Today, no mechanomyographic device is commercially available. In clinical settings the most common quantitative monitoring device is AMG. It measures the acceleration of the thumb with a piezo-electric wafer fixed on the distal phalanx. Since the acceleration is linearly related to the contraction force due to Newton’s second law, AMG is a mechanical method. AMG data have an idiosyncrasy of unknown reason, i.e. the control TOFR is commonly >1.0, sometimes up to 1.4 and even higher.19 Therefore, AMG devices additionally require normalization of the TOFR to overcome this issue, i.e. the TOFR has to recover to at least 90% of the baseline TOFR measured before giving a neuromuscular blocking drug.19,20 Since normalization is not included in the software of any acceleromyograph, anesthesiologists must do it manually.19 Unfortunately, some AMG devices do not display raw TOFR values. Some limit the displayed values to 1.0, others switch their TOFR calculation at T4/T1 > 1.0 to T4/T2.21 Irrespectively, reliance on AMG may be limited by any of these approaches. Further limitations are related to the need for a stable preload, free mobility of the thumb, and fixation of the hand. New triaxial or 3-dimensional transducers may resolve some of these limitations but have still not been validated against EMG or mechanomyography. EMG is less common although it is considered as an alternative gold standard due to its high correlation with mechanomyography.22 The electrical twitch response of muscle measured with EMG is not affected by changes in muscle contractility. Therefore, the thumb needs neither preload, nor free mobility, and the hand needs no immobilization. Furthermore, the electric twitch response seems to be less sensitive to hyperthermia than muscle contraction.23
Clinical assessment of neuromuscular function
Despite the overwhelming evidence of the benefits of neuromuscular monitoring, many anesthesia providers still use less reliable methods for evaluating neuromuscular function in anesthetized or recently extubated and uncooperative patients. The ability to lift the head, a firm handshake or a sufficient minute ventilation of an intubated patient are often erroneously misinterpreted as adequate recovery from neuromuscular block. Patients can have normal tidal volume despite a TOFR below 0.1, even normal vital capacity despite a TOFR = 0.6,24 and can lift their heads for 5 seconds at a TOFR = 0.3.25 Clinical tests used to detect residual neuromuscular block should therefore not be considered an option in patient-safety focused anesthesia.
Despite the overwhelming evidence of the benefits of neuromuscular monitoring, many anesthesia providers still use less reliable methods for evaluating neuromuscular function
Definition of residual neuromuscular block
The first threshold for acceptable recovery was set at a TOFR > 0.7 based on a study in healthy volunteers, who had normal vital capacity, inspiratory force, and peak expiratory flow rate at that value.24 Two decades later the level of acceptable recovery had to be increased to 0.9 based on several observations demonstrating subjective weakness,26 decreased hypoxic ventilatory response,9 impaired protective airway reflexes,27,28 and upper airway obstruction29 at TOFRs between 0.7 and 0.9. More recently it was suggested that a TOFR of unity might be even better, especially if neuromuscular function is monitored by AMG.8
Incidence of residual neuromuscular block
In one of the first published reports on post-operative residual paralysis, Viby-Mogensen and colleagues found an incidence of 42% of patients with TOFR < 0.7.30 When some years later the level of sufficient recovery was set to TOFR ≥ 0.9, the incidence was up to 83%,31 but varies greatly amongst studies depending on the choice of muscle relaxant,12,31,32 the technique of neuromuscular monitoring,33 the use of reversal drugs,32,34 the duration of surgery,34,35 and the amount of incremental doses.36,37 Older age appears to be a risk factor of residual NMBA; a recent study conducted in the USA reported an incidence of residual NMBA in the PACU even after administration of intermediate acting NMBA of 57.7% in elderly patients.38
Reversal of neuromuscular block
There are two options to reach a sufficient TOFR > 0.9: wait for spontaneous recovery or pharmacological reversal. Waiting until the patients’ neuromuscular function has recovered spontaneously is a safe but seldom used option. Reversal can be done either by acetylcholine esterase inhibitors, such as pyridostigmine or neostigmine or by sugammadex. Acetylcholine esterase inhibitors increase the amount of acetylcholine in the synaptic cleft by inhibition of its hydrolysis. Complete inhibition of the acetylcholine esterase results in a maximally increased acetylcholine concentration in the synaptic cleft. If this concentration is not sufficient to counteract the competitive inhibition by the muscle relaxant, a residual neuromuscular block is still present. Increments of the acetylcholine esterase inhibitor do not act (ceiling effect). Therefore, acetylcholine esterase inhibitors are effective to reverse shallow or minimal residual blocks only (Table 1).39-42 Table 1: Depth of block according to the consensus statement of neuromuscular monitoring22 and dose recommendations according to several dose finding studies for neostigmine39-42 and sugammadex39,40,43,44. Qualitative neuromuscular monitoring cannot determine a TOFR. In these patients the lowest dose of sugammadex is 1 mg/kg and for neostigmine 40 µg/kg.
Quantitative neuromuscular monitoring is the only way to detect and quantify residual neuromuscular blocks.
*These doses have been determined in dose-finding studies, were however not tested in comparative clinical trials. They are not recommended by the manufacturer. Please note that the use of low-dose sugammadex has been questioned, since elderly patients are at greater risk for recurarization and residual muscle paralysis when low-dose sugammadex is administered. Sugammadex, a modified cyclodextrine, encapsulates steroidal muscle relaxants in a 1:1 relation. The complex is renally excreted. Sugammadex is, therefore, able to reverse any depth of neuromuscular block.39,40,43,44,46,47 Sugammadex is dosed according to the degree of residual neuromuscular block (see Table 1).
How to avoid residual neuromuscular block
Every drug should only be given if necessary. Accordingly, the easiest strategy to avoid residual neuromuscular blocks, therefore, is to avoid using muscle relaxants. If ventilation of the patient can be achieved with a supraglottic airway device, which does not require a muscle relaxant for placement, this technique should be preferred.13 Furthermore, not every operation necessitates a neuromuscular block. If omission of muscle relaxants is not a solution for a particular patient or surgery, anesthetists need a method to avoid residual neuromuscular blockade. Therefore, titrating the depth of the neuromuscular block based on surgical needs is increasingly recognized as an appropriate methodology in these patients. By doing so, however, we recommend a strategy to avoid a residual neuromuscular block based on quantitative neuromuscular monitoring during the complete course of anesthesia - from induction to emergence - and reversal of a residual neuromuscular block if necessary. Based on published data we developed a feasible clinical algorithm to minimize residual neuromuscular block while maintaining the current workflow in the operating room.48 The clinical algorithm makes the following assumptions:
- Muscle relaxants are a component of a modern balanced anesthesia.
- Every depth of neuromuscular block by every neuromuscular blocking agent is included.
- Undetected and untreated residual neuromuscular blocks are unpleasant and potentially harmful for the patient.
- Reversal drugs have side effects. Therefore, there is the need for a clear indication and appropriate dosing.
- The effects of reversal drugs must be controlled.
- Since emergence from anesthesia takes roughly 10 minutes, the algorithm to avoid residual neuromuscular block has to work within this time frame.
Algorithm to avoid residual neuromuscular blocks based on quantitative neuromuscular monitoring (Figure 1)
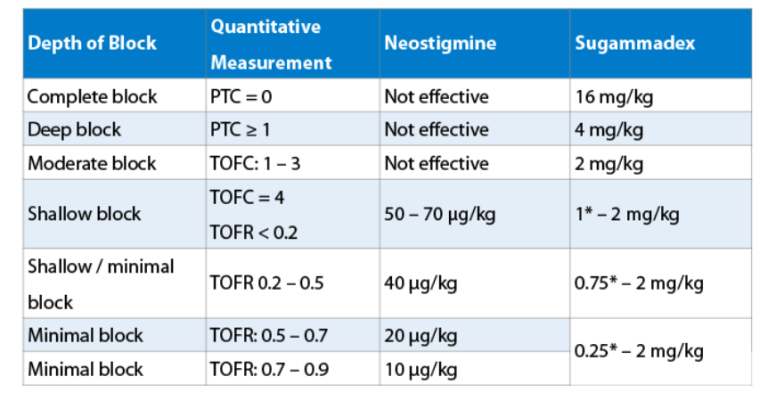
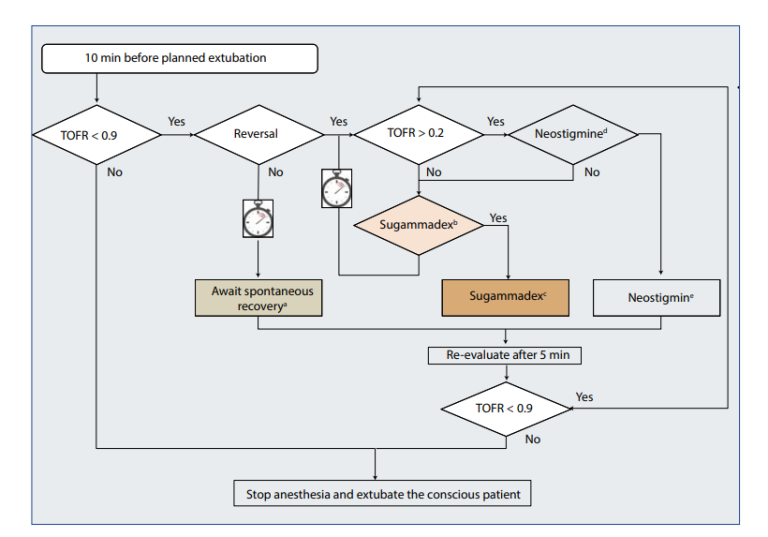
Await spontaneous recovery. At a TOFR < 0.5, the goal to reverse a residual block within 10 minutes will most probably not met. Depending on local and organizational circumstances spontaneous recovery can be awaited in the OR, induction room, PACU, or intensive care unit. Check indication for sugammadex. Aside from proof of neuromuscular block consider:
-
contraindications for sugammadex
-
sugammadex is only suitable for reversing steroidal muscle relaxants Sugammadex doses for quantitative neuromuscular monitoring (see Table 1) check indication for neostigmine. Aside from proof of neuromuscular block consider:
-
contraindications for neostigmine
-
maximum dose for neostigmine is 70 µg/kg
-
little effect in reversing mivacurium
Quantitative neuromuscular monitoring is the only way to detect and quantify residual neuromuscular blocks. At a TOFR above 0.9 the patient has sufficiently recovered from his neuromuscular block and in most cases can be safely extubated. At TOFR ≤ 0.9, residual neuromuscular blockade is present and has to be treated. This can be done either by simply waiting or giving the patient a reversal drug. At a TOFR < 0.5 spontaneous recovery will delay extubation for at least 10 minutes.40 Drug and dose when opting for pharmacological reversal is dependent on the measured TOFR and the used neuromuscular blocking agent. Because of the ceiling effect, reversal with neostigmine is recommended at a TOFR > 0.2 (Table 1). If neuromuscular recovery is too slow within the first 5 minutes, an additional dose of neostigmine up to the maximum dose of 70 µg/kg can be considered to achieve maximum inhibition of cholinesterase.49 If the TOFR is below 0.2 at the time of reversal or if contraindications against neostigmine are present, sugammadex can reverse any rocuronium or vecuronium induced neuromuscular block (Table 1). Reversal with sugammadex is also possible in case of incomplete recovery after neostigmine.50 Importantly, complete recovery has to be controlled for at least 5 min.
Comparative assessment of the algorithm
The goal of the proposed algorithm is to avoid any residual neuromuscular block with therapeutic concepts based on neuromuscular monitoring and a rational use of muscle relaxant reversers. Neuromuscular monitoring by itself, however, does not reduce the complications of residual neuromuscular block.13,15 On the other hand, without neuromuscular monitoring, anesthetists do not have a rational base to decide which reversal agent and which dose is appropriate. Furthermore, without neuromuscular monitoring an area of uncertainty remains after administration of reversal agents, which cannot be solved with routine or liberal administration of reversal agents.51 The algorithm allows the perception of any residual neuromuscular block, especially those with TOFR between 0.4 and 0.9. Additionally, quantitative monitoring allows dose adaptions of the reversal agents to the actual depth of block. Importantly, quantitative neuromuscular monitoring can indicate complete neuromuscular recovery with a TOFR > 0.9. The strength of quantitative neuromuscular monitoring is the proof of full recovery and the measurement of the degree of residual block. Taken together it allows the anesthetist to adapt the reversal drugs to the respective residual block, using neostigmine as well as Sugammadex (Table 1). Furthermore, quantitative neuromuscular monitoring indicates when neostigmine does not have a realistic chance to provide neuromuscular recovery within 10 min, i.e. at TOFR < 0.2 even at a dose of 70 µg/kg.52 It also allows significantly smaller doses of neostigmine of 10-20 µg/kg at residual blocks with TOFR > 0.5,41,42 as the success of this measure can be controlled and corrected if necessary.
The goal of the proposed algorithm is to avoid any residual neuromuscular block with therapeutic concepts based on neuromuscular monitoring and a rational use of muscle relaxant reversers.
The final remaining question is which neuromuscular monitoring technique should be preferred, especially since AMG seems to overestimate the degree of neuromuscular recovery.53 It has therefore been suggested to increase the acceleromyographic level of full recovery to a TOFR = 1.0.20 Efficient use of EMG, correctly placed, may greatly enhance the ability to guarantee complete recovery from neuromuscular block, to estimate the optimal dose of reversal agents, and to enhance patient throughput in a cost-effective manner Implementation of a new algorithm into clinical practice Healthcare administrators and hospital systems often implement new guidelines to improve physician practices and patient outcomes. However, the efficacies of these initiatives is often poor, since a cultural change needs to be achieved. Todd and colleagues reported the outcomes of a sustained educational effort, which included a series of surveys of residual neuromuscular block, case discussions and literature review over the course of two years following the introduction of quantitative TOF monitors to the operating room.54 Studies have shown that multifaceted approaches are superior to single interventions.55,56 We have recently demonstrated at the Massachusetts General Hospital in Boston that a bundled intervention to optimize the use of reversal agents improved relevant postoperative respiratory outcomes.57 The clinical setting in which anesthesia is administered offers the opportunity to use changes in the practice environment as a strategy to influence clinician behavior. Thus, we suggest based on our observations made in the USA, that any quality improvement such as the one discussed in this article needs to be locally discussed, probably somewhat modified, and rigorously implemented.58
Conclusion
Residual neuromuscular block poses an unnecessary threat to patients. The basic principle for preventing residual neuromuscular block is quantitative neuromuscular monitoring. Three methods of neuromuscular monitoring have been addressed in this paper, including AMG, KMG and EMG. All techniques must be calibrated to increase the reliability of the measurement. In clinical settings, the most common quantitative monitoring device is AMG, however while EMG is less common, it is considered as an alternative gold standard due to its high agreement with mechanomyography. However, neither neuromuscular monitoring nor reversal agents by themselves can prevent residual neuromuscular block. A combination of both neuromuscular monitoring and reversal agents, in terms of an algorithm can reduce incidence and severity of this unwanted anesthetic complication. We provided an algorithm using quantitative neuromuscular monitoring, to help reliably detect and reverse a possible residual neuromuscular block depending on the clinical situation. In clinical settings, the most common quantitative monitoring device is AMG, however while EMG is less common, it is considered as an alternative gold standard due to its high agreement with mechanomyography.
References
1. Lundstrom LH, Duez CHV, Norskov AK, Rosenstock CV, Thomsen JL, Moller AM, Strande S, Wetterslev J. Effects of avoidance or use of neuromuscular blocking agents on outcomes in tracheal intubation: a Cochrane systematic review. Br J Anaesth 2018;120:1381-93.
2. Mencke T, Echternach M, Kleinschmidt S, Lux P, Barth V, Plinkert PK, Fuchs-Buder T. Laryngeal morbidity and quality of tracheal intubation: a randomized controlled trial. Anesthesiology 2003;98:1049-56.
3. Torensma B, Martini CH, Boon M, Olofsen E, In ‘t Veld B, Liem RS, Knook MT, Swank DJ, Dahan A. Deep Neuromuscular block improves surgical conditions during bariatric surgery and reduces postoperative pain: a randomized double blind controlled trial. PLoS One 2016;11:e0167907.
4. Blobner M, Frick CG, Stauble RB, Feussner H, Schaller SJ, Unterbuchner C, Lingg C, Geisler M, Fink H. Neuromuscular blockade improves surgical conditions (NISCO). Surg Endosc 2015;29:627-36.
5. Veelo DP, Gisbertz SS, Binnekade JM, Hannivoort RA, Bosman JA, Geerts BF, Blobner M, van Berge Henegouwen MI, Hollmann MW. On-demand versus continuous rocuronium infusion for deep neuromuscular relaxation in patients undergoing thoraco-laparoscopic esophagectomy: a randomized-controlled clinical trial (DEPTH). Can J Anaesth 2019.
6. Rosenberg J, Herring WJ, Blobner M, et al. Deep Neuromuscular Blockade Improves Laparoscopic Surgical Conditions: A Randomized, Controlled Study. Adv Ther 2017;34:925-36.
7. Herbstreit F, Peters J, Eikermann M. Impaired upper airway integrity by residual neuromuscular blockade: increased airway collapsibility and blunted genioglossus muscle activity in response to negative pharyngeal pressure. Anesthesiology 2009;110:1253-60.
8. Eikermann M, Groeben H, Husing J, Peters J. Accelerometry of adductor pollicis muscle predicts recovery of respiratory function from neuromuscular blockade. Anesthesiology 2003;98:1333-7.
9. Eriksson LI, Sato M, Severinghaus JW. Effect of a vecuronium-induced partial neuromuscular block on hypoxic ventilatory response. Anesthesiology 1993;78:693-9.
10. Murphy GS, Szokol JW, Marymont JH, Greenberg SB, Avram MJ, Vender JS. Residual neuromuscular blockade and critical respiratory events in the postanesthesia care unit. Anesth Analg 2008;107:130-7.
11. Bissinger U, Schimek F, Lenz G. Postoperative residual paralysis and respiratory status: a comparative study of pancuronium and vecuronium. Physiol Res 2000;49:455-62.
12. Berg H, Roed J, Viby-Mogensen J, Mortensen CR, Engbaek J, Skovgaard LT, Krintel JJ. Residual neuromuscular block is a risk factor for postoperative pulmonary complications. A prospective, randomised, and blinded study of postoperative pulmonary complications after atracurium, vecuronium and pancuronium. Acta Anaesthesiol Scand 1997;41:1095-103.
13. Kirmeier E, Eriksson LI, Lewald H, Jonsson Fagerlund M, Hoeft A, Hollmann M, Meistelman C, Hunter JM, Ulm K, Blobner M. Post-anaesthesia pulmonary complications after use of muscle relaxants (POPULAR): a multicentre, prospective observational study. The Lancet Respiratory Medicine 2019;7:129-40.
14. Bulka CM, Terekhov MA, Martin BJ, Dmochowski RR, Hayes RM, Ehrenfeld JM. Nondepolarizing neuromuscular blocking agents, reversal, and risk of postoperative pneumonia. Anesthesiology 2016;125:647-55.
15. Grosse-Sundrup M, Henneman JP, Sandberg WS, Bateman BT, Uribe JV, Nguyen NT, Ehrenfeld JM, Martinez EA, Kurth T, Eikermann M. Intermediate acting non-depolarizing neuromuscular blocking agents and risk of postoperative respiratory complications: prospective propensity score matched cohort study. BMJ 2012;345:e6329.
16. Arbous MS, Meursing AEE, van Kleef JW, de Lange JJ, Spoormans HHAJM, Touw P, Werner FM, Grobbee DE. Impact of anesthesia management characteristics on severe morbidity and mortality. Anesthesiology 2005;102:257-68; quiz 491-2.
17. Viby-Mogensen J, Jensen NH, Engbaek J, Ording H, Skovgaard LT, Chraemmer-Jorgensen B. Tactile and visual evaluation of the response to train-of-four nerve stimulation. Anesthesiology 1985;63:440-3.
18. Fuchs-Buder T, Claudius C, Skovgaard LT, Eriksson LI, Mirakhur RK, Viby-Mogensen J, th International Neuromuscular M. Good clinical research practice in pharmacodynamic studies of neuromuscular blocking agents II: the Stockholm revision. Acta Anaesthesiol Scand 2007;51:789-808.
19. Suzuki T, Fukano N, Kitajima O, Saeki S, Ogawa S. Normalization of acceleromyographic train-of-four ratio by baseline value for detecting residual neuromuscular block. Br J Anaesth 2006;96:44-7.
20. Capron F, Alla F, Hottier C, Meistelman C, Fuchs-Buder T. Can acceleromyography detect low levels of residual paralysis? A probability approach to detect a mechanomyographic train-of-four ratio of 0.9. Anesthesiology 2004;100:1119-24.
21. Murphy GS, Szokol JW, Avram MJ, Greenberg SB, Shear TD, Deshur M, Benson J, Newmark RL, Maher CE. Comparison of the TOFscan and the TOF-Watch SX during Recovery of neuromuscular function. Anesthesiology 2018;129:880-8.
22. Naguib M, Brull SJ, Kopman AF, Hunter JM, Fulesdi B, Arkes HR, Elstein A, Todd MM, Johnson KB. Consensus Statement on perioperative Use of neuromuscular monitoring. Anesth Analg 2018;127:71-80.
23. Kopman AF, Justo MD, Mallhi MU, Abara CE, Neuman GG. The influence of changes in hand temperature on the indirectly evoked electromyogram of the first dorsal interosseous muscle. Can J Anaesth 1995;42:1090-5
24. Ali HH, Wilson RS, Savarese JJ, Kitz RJ. The effect of tubocurarine on indirectly elicited train-of-four muscle response and respiratory measurements in humans. Br J Anaesth 1975;47:570-4.
25. Unterbuchner C, Blobner M, Pühringer F, Janda M, Bischoff S, Bein B, Schmidt A, Ulm K, Pithamitsis V, Fink H. Development of an algorithm using clinical tests to avoid post-operative residual neuromuscular block. BMC Anesthesiology 2017;17:101.
26. Kopman AF, Yee PS, Neuman GG. Relationship of the train-of-four fade ratio to clinical signs and symptoms of residual paralysis in awake volunteers. Anesthesiology 1997;86:765-71.
27. Sundman E, Witt H, Olsson R, Ekberg O, Kuylenstierna R, Eriksson LI. The incidence and mechanisms of pharyngeal and upper esophageal dysfunction in partially paralyzed humans: pharyngeal videoradiography and simultaneous manometry after atracurium. Anesthesiology 2000;92:977- 84.
28. Eriksson LI, Sundman E, Olsson R, Nilsson L, Witt H, Ekberg O, Kuylenstierna R. Functional assessment of the pharynx at rest and during swallowing in partially paralyzed humans: simultaneous videomanometry and mechanomyography of awake human volunteers. Anesthesiology 1997;87:1035-43.
29. Eikermann M, Vogt FM, Herbstreit F, Vahid-Dastgerdi M, Zenge MO, Ochterbeck C, de Greiff A, Peters J. The predisposition to inspiratory upper airway collapse during partial neuromuscular blockade. Am J Respir Crit Care Med 2007;175:9-15.
30. Viby-Mogensen J, Chraemer Jorgensen B, Ording H. Residual Curarization in the Recovery Room. Anesthesiology 1979;50:539 - 41.
31. Murphy GS, Szokol JW, Franklin M, Marymont JH, Avram MJ, Vender JS. Postanesthesia care unit recovery times and neuromuscular blocking drugs: a prospective study of orthopedic surgical patients randomized to receive pancuronium or rocuronium. Anesth Analg 2004;98:193-200, table of contents.
32. Martinez-Ubieto J, Ortega-Lucea S, Pascual-Bellosta A, Arazo-Iglesias I, Gil-Bona J, Jimenez-Bernardo T, Munoz-Rodriguez L. Prospective study of residual neuromuscular block and postoperative respiratory complications in patients reversed with neostigmine versus sugammadex. Minerva Anestesiol 2016;82:735-42.
33. Murphy GS, Szokol JW, Marymont JH, Greenberg SB, Avram MJ, Vender JS, Nisman M. Intraoperative acceleromyographic monitoring reduces the risk of residual neuromuscular blockade and adverse respiratory events in the postanesthesia care unit. Anesthesiology 2008;109:389-98.
34. Stewart PA, Liang SS, Li QS, Huang ML, Bilgin AB, Kim D, Phillips S. The impact of residual neuromuscular blockade, oversedation, and hypothermia on adverse respiratory events in a postanesthetic care unit: a prospective study of prevalence, predictors, and outcomes. Anesth Analg 2016;123:859-68.
35. Debaene B, Plaud B, Dilly M-P, Donati F. Residual paralysis in the PACU after a single intubating dose of nondepolarizing muscle relaxant with an intermediate duration of action. Anesthesiology 2003;98:1042-8.
36. Maybauer DM, Geldner G, Blobner M, Puhringer F, Hofmockel R, Rex C, Wulf HF, Eberhart L, Arndt C, Eikermann M. Incidence and duration of residual paralysis at the end of surgery after multiple administrations of cisatracurium and rocuronium. Anaesthesia 2007;62:12-7.
37. Fortier L-P, McKeen D, Turner K, de Médicis É, Warriner B, Jones PM, Chaput A, Pouliot J-F, Galarneau A. The RECITE Study: A Canadian prospective, multicenter study of the incidence and severity of residual neuromuscular blockade. Anesthesia and Analgesia 2015;121:366-72.
38. Murphy GS, Szokol JW, Avram MJ, Greenberg SB, Shear TD, Vender JS, Parikh KN, Patel SS, Patel A. Residual Neuromuscular Block in the Elderly: Incidence and Clinical Implications. Anesthesiology 2015;123:1322-36.
39. Kaufhold N, Schaller SJ, Stauble CG, Baumuller E, Ulm K, Blobner M, Fink H. Sugammadex and neostigmine dose-finding study for reversal of residual neuromuscular block at a train-of-four ratio of 0.2 (SUNDRO20)dagger. Br J Anaesth 2016;116:233-40.
40. Schaller SJ, Fink H, Ulm K, Blobner M. Sugammadex and neostigmine dose-finding study for reversal of shallow residual neuromuscular block. Anesthesiology 2010;113:1054-60.
41. Fuchs-Buder T, Baumann C, De Guis J, Guerci P, Meistelman C. Low-dose neostigmine to antagonise shallow atracurium neuromuscular block during inhalational anaesthesia: A randomised controlled trial. Eur J Anaesthesiol 2013;30:594-8.
42. Fuchs-Buder T, Meistelman C, Alla F, Grandjean A, Wuthrich Y, Donati F. Antagonism of low degrees of atracurium-induced neuromuscular blockade: dose-effect relationship for neostigmine. Anesthesiology 2010;112:34-40.
43. Sorgenfrei I, Norrild K, Larsen P, Stensballe J, Ostergaard D, Prins M, Viby-Mogensen J. Reversal of rocuronium-induced neuromuscular block by the selective relaxant binding agent sugammadex. A dose-finding and safety study. Anesthesiology 2006;104:667-74.
44. Puhringer FK, Rex C, Sielenkamper AW, Claudius C, Larsen PB, Prins ME, Eikermann M, Khuenl-Brady KS. Reversal of profound, high-dose rocuronium-induced neuromuscular blockade by sugammadex at two different time points: an international, multicenter, randomized, dose-finding, safety assessor-blinded, phase II trial. Anesthesiology 2008;109:188-97.
45. Muramatsu T, Isono S, Ishikawa T, Nozaki-Taguchi N, Okazaki J, Kitamura Y, Murakami N, Sato Y. Differences of recovery from rocuronium-induced deep paralysis in response to small doses of sugammadex between elderly and nonelderly patients. Anesthesiology 2018.
46. Jones RK, Caldwell JE, Brull SJ, Soto RG. Reversal of profound rocuronium-induced blockade with sugammadex: a randomized comparison with neostigmine. Anesthesiology 2008;109:816-24.
47. Herring WJ, Woo T, Assaid CA, Lupinacci RJ, Lemmens HJ, Blobner M, Khuenl-Brady KS. Sugammadex efficacy for reversal of rocuronium- and vecuronium-induced neuromuscular blockade: A pooled analysis of 26 studies. J Clin Anesth 2017;41:84-91.
48. Ripke F, Fink H, Blobner M. Concepts for the avoidance of residual neuromuscular blockades after the administration of nondepolarizing muscle relaxants. Anasth Intensivmed 2014;55:564-76.
49. Magorian TT, Lynam DP, Caldwell JE, Miller RD. Can early administration of neostigmine, in single or repeated doses, alter the course of neuromuscular recovery from a vecuronium-induced neuromuscular blockade? Anesthesiology 1990;73:410-4.
50. de Menezes CC, Peceguini LA, Silva ED, Simoes CM. Use of sugammadex after neostigmine incomplete reversal of rocuronium-induced neuromuscular blockade. Revista brasileira de anestesiologia 2012;62:543-7.
51. Kotake Y, Ochiai R, Suzuki T, Ogawa S, Takagi S, Ozaki M, Nakatsuka I, Takeda J. Reversal with sugammadex in the absence of monitoring did not preclude residual neuromuscular block. Anesth Analg 2013;117:345-51.
52. Harper NJN, Wallace M, Hall IA. Optimum Dose of Neostigmine at Two Levels of Atracurium-Induced Neuromuscular Block. BJ of Anaesthesia 1994;72:82-5.
53. Liang SS, Stewart PA, Phillips S. An ipsilateral comparison of acceleromyography and electromyography during recovery from nondepolarizing neuromuscular block under general anesthesia in humans. Anesth Analg 2013;117:373- 9.
54. Todd MM, Hindman BJ, King BJ. The implementation of quantitative electromyographic neuromuscular monitoring in an academic anesthesia department. Anesth Analg 2014;119:323-31.
55. Johnson MJ, May CR. Promoting professional behaviour change in healthcare: what interventions work, and why? A theory-led overview of systematic reviews. BMJ Open 2015;5:e008592.
56. Mostofian F, Ruban C, Simunovic N, Bhandari M. Changing physician behavior: What works? Am J Manag Care 2015;21:75-84.
57. Rudolph MI, Chitilian HV, Ng PY, Timm FP, Agarwala AV, Doney AB, Ramachandran SK, Houle TT, Eikermann M. Implementation of a new strategy to improve the peri-operative management of neuromuscular blockade and its effects on postoperative pulmonary complications. Anaesthesia 2018;73:1067-78.
58. Schmidt U, Eikermann M. Organizational aspects of difficult airway management: think globally, act locally. Anesthesiology 2011;114:3
Continuing Education (CME)
This activity has been planned and implemented in accordance with the accreditation requirements and policies of the Accreditation Council for Continuing Medical Education (ACCME) through the joint providership of SynAptiv and Saxe Healthcare Communications. SynAptiv is accredited by the ACCME to provide continuing medical education for physicians. SynAptiv designates this live activity for a maximum of 2 AMA PRA Category 1 Credit™. Physicians should claim only the credit commensurate with the extent of their participation in the activity. Continuing Education (CNE/CRCE) Saxe Healthcare Communications is accredited as a provider for continuing education. Provider approved by California Board of Nursing. Provider #14477 and the Florida Board of Nursing Provider # 50-17032 This program has been approved for 2.0 contact hours Continuing Respiratory Care Education (CRCE) credit by the American Association of Respiratory Care.
Learning Objectives
Upon completion of this activity, the participant should be able to:
- Discuss the use of neuromuscular monitoring for the prevention residual neuromuscular block during anesthesia.
- Describe the three (3) technologies of neuromuscular monitoring.
- Discuss the use of both neuromuscular monitoring and reversal agents in combination with an algorithm to reduce anesthetic complications.
To Obtain your CEs
Go to www.saxetesting.com/ge. You will need to register on the test site. Complete the evaluation form and the post-test. Upon successful submission, you will be able to print your certificate of completion