Abstract
Neuromuscular blocking agents (NMBAs) have a variety of indications for use in the ICU, but they come with the risk of side effects that can be severe. Accordingly, in order to ensure optimal dosing, it is important to monitor depth of neuromuscular blockade (NMB) in ICU patients being treated with NMBAs. While there are qualitative and quantitative methods of monitoring, studies have documented that quantitative methods are more reliable and effective.44 Patients could benefit from more widespread monitoring of NMB in the ICU. More data is needed to support its wider adoption in the United States; clinicians must understand the paramount importance of quantitative NMB monitoring and receive adequate education.
Overview of Neuromuscular Blocking Agents
Neuromuscular blocking agents (NMBAs) cause skeletal muscle relaxation via interference of neurochemical transmissions at the neuromuscular junction. NMBAs target the chemical exchange that occurs between the end of a neuron and the muscle fiber or the neuromuscular junction (Figure 1). During regular neuronal stimulation, an action potential travels to the motor terminus, and subsequent depolarization leads to release of a neurotransmitter, acetylcholine, which interfaces with postsynaptic nicotinic receptors located on the muscle. This results in an excitatory postsynaptic end-plate potential that produces muscle contraction. NMBAs interfere with this physiologic procedure, either by binding to the postsynaptic receptors and causing depolarization of the muscle (depolarizing agents such as succinylcholine), or by competitively binding to postsynaptic receptors to prevent acetylcholine binding and action, without depolarizing the muscle (nondepolarizing agents such as rocuronium).
Figure 1: Depicts the major components of the neuromuscular junction: the neuron, acetylcholine, and the muscle. Acetylcholine is stored in vesicles within the neuron. When an action potential is generated, acetylcholine is released into the synapse in a process called exocytosis. Acetylcholine binds to receptors on the muscle cell to simulate muscle contraction.
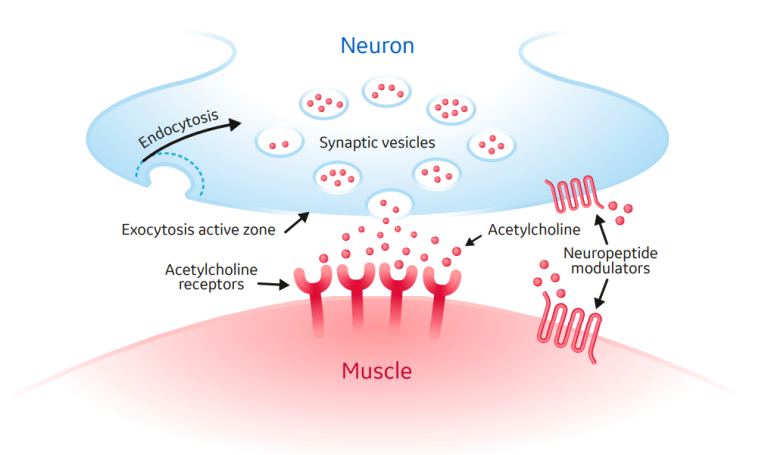
Figure 2: Subtypes of neuromuscular blocking agents
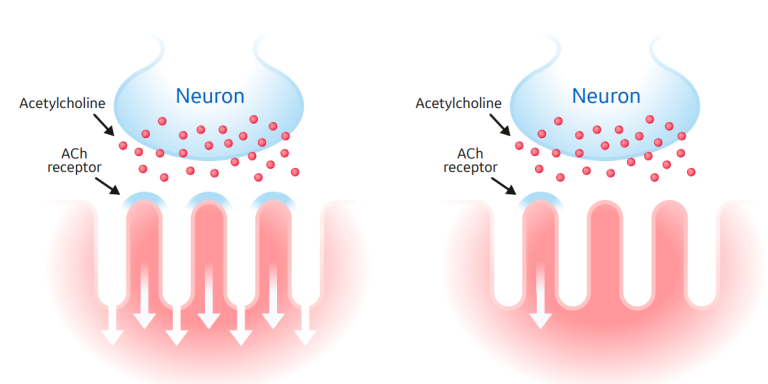
NMBAs are separated into two subtypes by their mechanism of action: depolarizing agents and non-depolarizing agents (Figure 2). These can be further subdivided by their duration of action--short, intermediate, and long acting. The only depolarizing agent available to clinicians is succinylcholine, which exerts its muscle relaxation effect by directly binding to acetylcholine receptors, causing continuous depolarization.1
By contrast, nondepolarizing agents competitively antagonize the nicotinic receptor. This action causes muscle relaxation by preventing acetylcholine from binding and depolarizing the muscle.2 Finally, nondepolarizing agents can further be divided by their chemical structure: benzylisoquinoliniums and aminosteroids. Each NMBA has a unique clinical profile, and ultimately the selection of a specific NMBA depends on patient characteristics, indication, and interactions with other drugs (Table 1).
Table 1: Pharmacokinetics & Drug Information on the Commonly used Neuromuscular Blocking Agents in the ICU
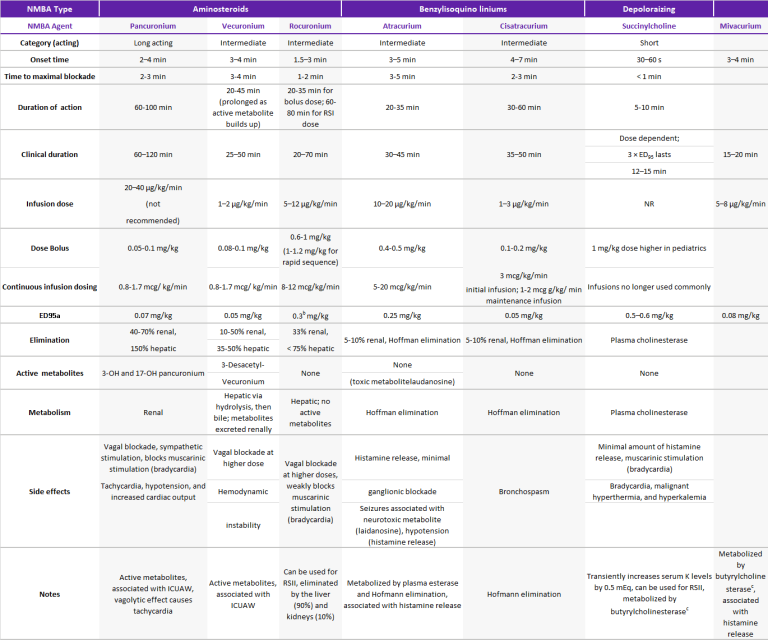
ED95: effective dose that decreases the twitch by 95% from baseline, ICUAW: intensive care unit-acquired weakness, NR: not recommended, RSII: rapid sequence induction and intubation, a : Intubating dose is 2 × ED95, b : 1.2 mg/kg (4 × ED95) can be used for rapid sequence induction and intubation, c : Also referred to as plasma cholinesterase or pseudocholinesterase, min: minute, NA: not applicable, RSI: rapid sequence induction. Source: References 5-7, 12, 25, 31, 32. Adapted from Sturgess et al. A Surgeon’s Guide to Anaesthesia and Peri-operative Care, 2014.
Indications for NMBAs in the Intensive Care Unit
NMBAs are frequently administered in the Intensive Care Unit (ICU). Indications include urgent intubations, acute respiratory distress syndrome (ARDS) (Paragraph 1), status asthmaticus (Paragraph 2), increased intracranial pressure (ICP), increased intra-abdominal pressure (IAP) (Paragraph 3), and therapeutic hypothermia after out of-hospital VF cardiac arrest.3,4
In the ICU, tracheal intubation is a life-saving intervention for patients with current or impending respiratory failure. NMBAs are an important adjunct for laryngoscopy and can decrease airway trauma during intubation.5 NMBA usage in ARDS has been shown to reduce barotrauma and improve oxygenation. However, there are conflicting studies reporting the risk/benefit of routine implementation.
Contemporary evidence suggests that treatment should be tailored on an individualized basis and directed in part by institutional protocols.3,4 Ventilator asynchrony remains among the most compelling indications for NMBA usage in the critical care setting. Eliminating patient respiratory effort with NMBA introduces a host of issues but is an effective mechanism to deliver desired settings on ventilators and reduce asynchrony, barotrauma and lung hyperinflation. Further, NMBAs are indicated in critical patients with elevated ICP that is refractory to conservative management (Paragraph 4). Sedation alone is often inadequate in lowering ICP, and NMBAs can mitigate the elevations in ICP from coughing, bucking or movement. Again, conflicting evidence prevents its universal adoption for reduction of ICP in all critical care settings.3,4 Lastly, in patients with out-of-hospital cardiac arrest, NMBAs are indicated for therapeutic hypothermia to alleviate shivering and improve survival.3,4
Current guidelines for ARDS treatment are considered for patients with a PaO2/FiO2 ratio of less than 150 mmHg in order to reduce mortality, and it should be administered via continuous infusion early for no more than 48 hours with daily evaluation.6
The advent of the COVID-19 pandemic has seen a significant increase in NMBA utilization. COVID-19 ARDS patients are frequently treated with NMBA which urges the need for further evaluation of this treatment modality. In a recent multicenter study conducted in 21 ICUs from France and Belgium, NMBA usage was monitored in 407 COVID-19 patients with moderate to severe ARDS (Berline definition) to determine the rate and time to breathing without assistance after 28 days.7 The study found that there was an increased and prolonged usage of NMBA in COVID-19 ARDS patients.
Moreover, there are a number of benefits of NMBA usage in patients with COVID-19. Specifically, NBMAs can be helpful for intubation to minimize the risk of coughing and infecting healthcare workers, for promoting ventilator synchrony, for facilitating prone position maneuvering for managing refractory hypoxemia, and for improving patient outcomes during laparoscopic operations. However, even with these theoretical improvements with NMBA for COVID-19 patients, quantitative monitoring is still recommended by a recent panel of international experts, as leaving COVID-19 patients with residual weakness during extubation could have many consequences. Objective monitoring can minimize the risk of coughing during intubation as it confirms adequate paralysis; it is also helpful for proning procedures.8
Paragraph 1: the use of neuromuscular blocking agents for acute respiratory distress syndrome in the intensive care unit.
In the citation of Papazian et al 24, a RCT (n=340) in severe ARDS treated with NMBA regimen Cisatracurium infusion x 48 hr vs placebo, the conclusion of the study has been: improved adjusted 90-d survival (p = 0.04), increased ventilator-free days (p = 0.03), increased organ dysfunction-free days (p = 0.01), decreased barotrauma (p = 0.03).
In the citation of Arroliga et al 25, a retrospective (n=549) analysis on ALI/ARDS treated with NMBA Regimen
- High PEEP group, 45% had NMBA
- low PEEP group, 33% had NMBA
the study conclusion has shown no difference in 60-d mortality or length of mechanical ventilation.
In the citation of Forel et al 26 ,a RCT (n=36) in ARDS patients undergoing NMBA regimen Cisatracurium infusion x 48 hr vs placebo, the conclusion of the study has been: significant reduction in pulmonary/ systemic cytokines (p = 0.005, p = 0.04) and increased oxygenation (p < 0.001).
In the citation of Arroliga et al 27 , a retrospective study (n=5183) in patients undergoing mechanical ventilation >=12 hours where 13% patients were given neuromuscular blocking agents, the conclusion has been: significant increase in ICU mortality (p < 0.001) and duration of mechanical ventilation (p < 0.001) and ICU length of stay (p < 0.001).
In the citation of Gainnier et al 28, a RCT (n=56) in severe ARDS treated with NMBA regimen Cisatracurium infusion x 48 hr vs placebo, the conclusion of the study has been: improvement in oxygenation (p = 0.021), decrease in FiO2 (p = 0.001), and decrease in PEEP (p = 0.036). In the citation of Lagneau et al 29, a RCT (n=102) in ARDS treated with NMBA regimen Cisatracurium 2 hours infusion guided by Train of Four, the conclusion of the study has been: increase in oxygenation in groups with NMBA (p = 0.0014).
In the citation of Conti et al 30, a prospective open label study (n=13) in ALI/ARDS treated with NMBA regimen 1 bolus Pancuronium, the conclusion of the study has been: no difference in oxygenation. In the citation of Bishop et al 31, a prospective open label study (n=9) in ALI/ARDS treated with NMBA regimen 1 bolus-Pancuronium, the conclusion of the study has been: no difference in oxygenation.
NMBA = neuromuscular blocking agent, RCT = randomized controlled trial, ALI = acute lung injury, ARDS = acute respiratory distress syndrome, PEEP = positive end-expiratory pressure.
Paragraph 2: the use of neuromuscular blocking agents for status asthmaticus in the intensive care unit
In the citation of Kesler et al 32, a retrospective (n=170) study where 67% patients received NMBA before 1995 and 77% patients received NMBA after 1995, the results of the study have shown no statistically significant difference in weakness between NMBA group and sedation-only group.
In the citation of Adnet et al 33, a retrospective (n=118) study where 54% patients received NMBA the results of the study have shown higher prevalence of muscle weakness, longer mechanical ventilation, longer ICU stay and increase prevalence of pneumonia in the NMBA group.
In the citation of Behbehani et al 34, a retrospective (n=86) study where 35% patients received NMBA the results of the study have shown increase myopathies with NMBAs.
In the citation of Leatherman et al 35, a retrospective (n=107) study where 65% patients received NMBA the results of the study have shown an increase prevalence of muscle weakness with steroids & NMBAs, but no increase in muscle weakness with use of benzylisoquinoliniums.
In the citation of Griffin et al 36, a case series (n=3) reviewed (n=18) case reports, where 100% patients received steroids and steroidal NMBA , the results of the study have shown all patients developed severe muscle weakness.
NMBA = neuromuscular blocking agent, ICU=Intensive Care Unit
Paragraph 3: studies involving neuromuscular blocking agent and intra-abdominal pressure.
In the citation of Cheatham et al 37, a prospective observational study (n=478) in which NMBAs were used with a combined medical/surgical management algorithm, an increased patient survival to hospital discharge from 50% to 72% (p=0.15) has been reported.
In the citation of Davies et al 38, a case report (n=1) were patients received Atracurium infusion x 48 hours , a reduced intra-abdominal pressure and provided good recovery with discharge at 12 days postoperatively has been reported.
In the citation of De Waele et al 39, case series (n=10) were patients received Cisatracurium bolus , the results of the study have shown that 9/10 patients had a reduced intra-abdominal pressure.
NMBA = neuromuscular blocking agent
Paragraph 4: the use of neuromuscular blocking agents in the neurointensive care unit.
In the citation of Schramm et al 40 ,a RCT (n=14) in neurosurgery, patients received Cisatracurium or Atracurium bolus and the results of the study have shown Atracurium-transient decrease in ICP, MAP, CPP, CBF, Cisatracurium, no effect on MAP, ICP, CPP, CBF.
In the citation of Schramm et al 41, a prospective open label (n=24) study, patients received Cisatracurium bolus and the results of the study have shown no effect on MAP, ICP, CPP, CBF.
In the citation of Prielipp et al 42, a prospective open label (n=6) study, patients received Doxacurium bolus and infusion. The results of the study have shown no effect on MAP, ICP, no prolonged weakness.
In the citation of Hsiang et al 43, a retrospective study (n=514) in traumatic brain injury, NMBA were used in <50% of patients. The results of the study have shown decrease mortality with NMBA but increase prevalence of severely disabled patients, pneumonia, and ICU stay.
NMBA = neuromuscular blocking agent, ICP = intracranial pressure, MAP = mean arterial pressure, CPP = celebral perfusion pressure, CBF = celebral blood flow, ICU= intensive care unit
Table 2: Levels of neuromuscular block and objective/subjective evaluation
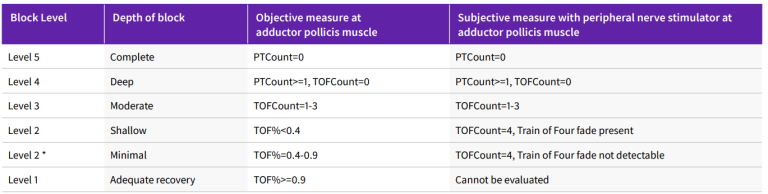
* Subjective evaluation of (minimal) depth of neuromuscolar block is not recommended. It could be considered a transition from current subjective evaluation practice to the most preferred objective monitoring-based practice.
PTC = posttetanic count, TOF = train of four, TOFC = train-of-four count.
Adapted from Renew, J.R., Ratzlaff, R., Hernandez-Torres, V. et al. Neuromuscular blockade management in the critically Ill patient. j intensive care 8, 37 (2020)
Why Patients Should Have Their NMB Level Monitored in the ICU
As with any therapy, NMBA use is associated with risks, especially with administration of higher dosages and longer durations and, in particular, in the absence of blockade depth monitoring (Table 6). Convention reinforces the notion that medications should be administered at a clinically effective dose without exceeding therapeutic doses.
Overdosing patients with NMBAs carries tremendous risk and exposes patients to untoward side effects. It is imperative to conduct clinical, qualitative, and quantitative evaluation and monitoring of the depth of neuromuscular blockade to ensure that the minimum effective dose is being administered.
Monitoring the depth of neuromuscular blockade allows for individualized titration of NMBA and results in a net reduction of NMBA dosage and duration. This in turn reduces the risk of complications related to NMBAs and is essential to avoiding prolonged paralysis in the ICU.
In 2015, the Association of Anesthetists of Great Britain and Ireland released guidelines for standards of monitoring anesthesia and recovery. The guidelines stated that a neuromuscular blockade monitoring device is essential for the conduct of anesthesia.9 Moreover, before extubation, a train-of-four (TOF) ratio above 0.9 is considered adequate to signal recovery from neuromuscular blockade.10 Therefore, neuromuscular monitoring is imperative to ensure recovery from neuromuscular blockade.
Despite this importance, studies continue to show a lack of utilization of monitoring systems in the United States, where 10% of anesthesiologists never use nerve stimulation for neuromuscular blockade monitoring.11,12 Similarly, nerve stimulation is used in less than 40% of patients and objective monitoring is used in less than 17% of patients to determine the depth of neuromuscular blockade.13,14 There are a limited number of studies that examine the significant clinical benefit of neuromuscular blockade monitoring for ICU patients; however, there are benefits to the patient and to the hospital in reducing the amount and duration of NMBAs in the ICU.
Risks of Neglecting to Monitor Depth of Neuromuscular Blockade
There are several risks associated with NMBA. Awareness during paralysis is one such complication that must be avoided. Ballard et. al. interviewed patients for their recollections during therapeutic paralysis in the ICU and found that patients with awareness during paralysis can have negative experiences during neuromuscular blockade; in these cases decreasing NMBA usage would be useful.15 Here, monitors are recommended to appropriately titrate NMBAs, as current protocols are not adequate.3
Residual neuromuscular blockade is another complication that is not routinely monitored in the ICU, and a recent case report by Workum et. al. showcases the complexities of NMBA usage in that setting. This underscores the recommendation to monitor TOF levels if continuous NMBA is used in the ICU both during and after cessation of continuous NMBA administration.16
Complications of residual weakness are associated with excess NMBA administration; these include difficulty swallowing, rapid shallow breathing, nasal flaring, overuse of accessory muscles, aspiration, atelectasis, pneumonia, and acute respiratory failure.3
ICU-acquired weakness (ICU-AW) has also been associated with NMBA usage; development of this condition can lead to delayed withdrawal of mechanical ventilation, extended hospitalization, poor prognosis, limited mobility, and lowered quality of life.17 Several case reports have linked NMBA as a risk factor, but there is a paucity of welldesigned trials to confirm this association.3
Deep vein thromboses (DVT) can occur with NMBAs through prolonged stasis – and a recent study demonstrated that NMBA is the strongest predictor for development of ICU-related DVTs.3
Corneal abrasions are associated with NMBA paralysis because the blink reflex is abolished and eyelid closure is hampered. This can lead to drying, scarring, ulceration and infection of the cornea.3
Moreover, pressure ulcers, myopathies, nerve injuries, pulmonary complications, and anaphylaxis are associated with the use of NMBA in the ICU.3,4
Monitoring depth of neuromuscular blockade is one way to mitigate the complications associated with incomplete reversal of neuromuscular block. Paradoxically, NMBA monitoring is not routinely used in the ICU. Dabaene et. al. found that 45% of patients arrived in the recovery room with residual neuromuscular block with a TOF ratio of less than 0.9, and an editorial published in Anesthesiology concluded that high numbers of patients did not have adequate neuromuscular function on arrival in the recovery room.18 Without routine monitoring of NMBAs, the risks of NMBA in the ICU and in the OR continue to be pervasive. Monitoring of NMB depth can lead to decreased dosage and lower risks of these complications.
Is There a Need for Reversal Agents?
Figure 3: NMBA reversal agents by subtype/family
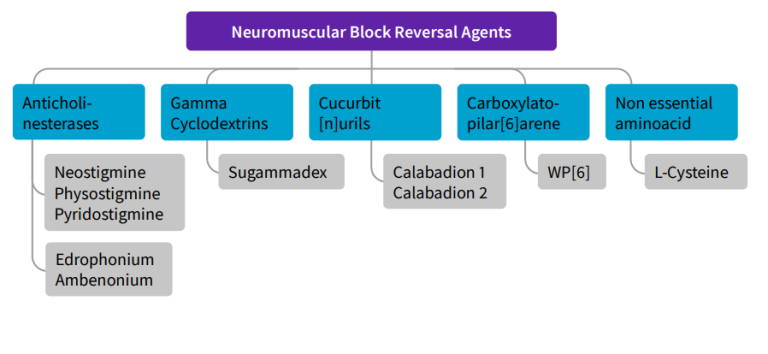
Reversal agents are used to restore baseline function through antagonism of NMBAs. This can quickly allow patients to recover from paralysis and reduce the risk of residual paralysis (Figure 3).
Anticholinesterase inhibitor drugs (i.e. neostigmine and edrophonium) function only with nondepolarizing agents; their mechanism is via inhibition of acetylcholinesterase and aggregate increases in acetylcholine at the neuromuscular junction. Due to the systemic increase in acetylcholine, antimuscarinics (glycopyrrolate) are also co-administered.
Sugammadex is a gamma-cyclodextrin compound that encapsulates and binds rocuronium and vecuronium. This action ultimately removes the NMBA from the neuromuscular junction for excretion in the urine. It has the ability to rescue deep blockade quickly; however, more research is necessary to support its safe and effective use in the ICU. Sugammadex is a dose-dependent drug, and the amount required to reverse deep blockade is four to eight times greater than for light blockade; underdosing may increase the risk for residual paralysis.19
Similarly, certain reversal agents are only effective at certain moments of paralysis, and neuromuscular transmission monitoring can be useful in deciding the dosage and timing of reversal agents in order to avoid complications such as residual paralysis.19 Currently, reversal agents are not routinely used in the ICU (spontaneous recovery is more common)4 and further studies are needed to validate their role in the ICU setting.3,4
Benefits of Quantitative vs. Qualitative Monitoring
A number of monitoring techniques and technologies have been developed to assess the depth of neuromuscular blockade. They are loosely classified as either qualitative or quantitative.
Qualitative methods
Physicians can first and foremost use clinical signs to assess recovery to baseline by using a variety of techniques, including presence of spontaneous ventilations, eye opening, sustained hand grip, leg lift, and sustained five second head lift. Unfortunately, these are poor determinants of residual neuromuscular blockade.
Peripheral Nerve Stimulation (PNS) or train-of-four (TOF) monitoring is a qualitative method in which four supramaximal electric pulses are administered every 0.5 seconds to the ulnar, facial, or posterior tibial nerve to generate up to four twitches. Clinicians can use the number of twitches to describe the depth of neuromuscular blockade.
Although PNS monitoring has improved recovery by reducing incidences of prolonged muscle paralysis and reduced overall NMBA administered, it is nevertheless insensitive for predicting residual neuromuscular weakness.3,4
These limitations include the subjective component of qualitative assessment; a recent study showed that even the most experienced clinicians had trouble discerning the fade of twitch response, which compares the strength of the fourth twitch to the first as a sign of depth of neuromuscular blockade.4 Other risks to PNS monitoring include loss of adhesion of the electrode to the skin, incorrect electrode placement, edema, and hypothermia.3
Lastly, different muscle groups have different sensitivities based on level of blood flow; this can further limit qualitative monitoring’s effectiveness in measuring level of neuromuscular blockade.20 For instance, facial muscles are more resistant to NMBAs, and so observations at this site can lead to overdosing.
Quantitative methods
Quantitative instruments objectively measure and confirm adequate recovery from NMBA via several methods. Acceleromyography (AMG) is the most commonly used; it applies Newton’s second law to measure the acceleration of the muscle group between the thumb and index finger. Kinemyography measures the degree of bending of the sensor strip between the thumb and index finger. Limitations of both systems require free movement of the hand. However, electromyography, another quantitative tool, does not require free movement of the hand. It also has a number of other advantages, including correctly estimating TOF ratios, less dependence on intraoperative normothermia than other mechanical technologies, and comparable accuracy to mechanomyography10 but more consistent in time.4
Quantitative monitoring is sparsely used in the ICU and other clinical environments. Although they are not widely adopted, many studies and panels of experts recommend its use in the ICU and OR and have found that routine use of quantitative monitors is the only reliable way to confirm recovery.4 Furthermore many societies, including the Czech republic Society in 2010 and New Zealand College of Anesthetists, have recommended the use of quantitative evaluation of blockade depth.10 Todd et. al. showed a significant reduction in the number of inadequately reversed patients arriving in the PACU of an academic anesthesia department if quantitative monitoring was used as opposed to qualitative methods.21 Quantitative monitoring may also result in net cost savings with less NMBA administered and reduction of complications.22
What is the Difference Between the OR and the ICU?
The OR and the ICU are different environments. The OR is a controlled environment, while the ICU is more unpredictable. The nuances between the two lead to differences in practice. For instance, the risk of a “failed” intubation is several times greater in the ICU than in the OR.23
Perioperative management in the OR lends itself to making clinical decisions that may be informed by data points frequently unavailable in the ICU. For example, several monitors may be pre-planned, such as incorporating invasive blood pressure monitoring by inserting an arterial line. In contrast, in the ICU, faster required responses from healthcare workers and more urgent demands detract from the ability to pre-plan. Other prominent differences include less availability of equipment to perform quantitative monitoring, and inadequate training to use quantitative monitors.
There is also a dearth of clinical evidence and guidelines/ recommendations for NMT monitoring in the ICU as compared to the OR. Paradoxically, while monitoring is less common in the ICU than in the OR, issues with monitoring are magnified in the ICU and arguably present as much risk to the patient.4
“What Can Be Done to Further Increase Adoption or Implementation of NMBA Monitoring in the ICU?”
Currently, quantitative monitoring is not routinely used in the ICU. There is also a paucity of well-designed studies that examine the use of NMBA monitoring – quantitative or otherwise – in the ICU. Overall, it will take an educational and cultural change in health care to implement NMBA monitors in the ICU. More data will be needed to support its adoption in the United States, and clinicians will need to be trained on its correct use and also the paramount utility of quantitative NMB monitoring. As with many quality initiatives, clinicians must embrace a culture shift that ultimately prioritizes patient safety over inertia and expediency.
Conclusion
NMBAs have many indications in the ICU, from facilitating endotracheal intubations to treating out-of-hospital cardiac arrest. However, as with any therapy, its usage can be associated with complications, including DVTs, ICU-AW, and residual paralysis. NMBA monitoring is a proven method that can be used to reduce the dosage and duration of NMBAs administered to a patient, and thus help clinicians prevent many complications and ultimately reduce costs and improve patient safety. Quantitative monitoring is considered the only method of reliably confirming recovery45 EMG technology offers many advantages over other qualitative methods of neuromuscular blockade monitoring
References
- Jonsson M, Dabrowski M, Gurley DA, et al. Activation and inhibition of human muscular and neuronal nicotinic acetylcholine receptors by succinylcholine. Anesthesiology. 2006;104(4):724-733. doi:10.1097/00000542-200604000-00017
- Jonsson Fagerlund M, Dabrowski M, Eriksson LI. Pharmacological characteristics of the inhibition of nondepolarizing neuromuscular blocking agents at human adult muscle nicotinic acetylcholine receptor. Anesthesiology. 2009;110(6):1244-1252. doi:10.1097/ ALN.0b013e31819fade3
- Greenberg SB, Vender J. The use of neuromuscular blocking agents in the ICU: where are we now? Crit Care Med. 2013;41(5):1332-1344. doi:10.1097/CCM.0b013e31828ce07c
- Renew JR, Ratzlaff R, Hernandez-Torres V, Brull SJ, Prielipp RC. Neuromuscular blockade management in the critically Ill patient. J intensive care. 2020;8:37. doi:10.1186/s40560-020-00455-2
- Wilcox SR, Bittner EA, Elmer J, et al. Neuromuscular blocking agent administration for emergent tracheal intubation is associated with decreased prevalence of procedure-related complications. Crit Care Med. 2012;40(6):1808-1813. doi:10.1097/CCM.0b013e31824e0e67
- Papazian L, Aubron C, Brochard L, et al. Formal guidelines: management of acute respiratory distress syndrome. Ann Intensive Care. 2019;9(1):69. doi:10.1186/s13613-019-0540-9
- Courcelle R, Gaudry S, Serck N, et al. Neuromuscular blocking agents (NMBA) for COVID-19 acute respiratory distress syndrome: a multicenter observational study. Crit Care. 2020;24(1):446. doi:10.1186/ s13054-020-03164-2
- Chaves-Cardona H, Hernandez-Torres V, Kiley S, Renew J. Neuromuscular blockade management in patients with COVID-19. Korean J Anesthesiol. 2021;74(4):285-292. doi:10.4097/kja.21106
- Checketts MR, Alladi R, Ferguson K, et al. Recommendations for standards of monitoring during anaesthesia and recovery 2015: Association of Anaesthetists of Great Britain and Ireland. Anaesthesia. 2016;71(1):85-93. doi:10.1111/anae.13316
- Duţu M, Ivaşcu R, Tudorache O, et al. Neuromuscular monitoring: an update. Rom J Anaesth intensive care. 2018;25(1):55-60. doi:10.21454/ rjaic.7518.251.nrm
- Naguib M, Kopman AF, Lien CA, Hunter JM, Lopez A, Brull SJ. A survey of current management of neuromuscular block in the United States and Europe. Anesth Analg. 2010;111(1):110-119. doi:10.1213/ ANE.0b013e3181c07428
- Hyman EC, Brull SJ. Clarification: Current Status of Neuromuscular Reversal and Monitoring, Challenges and Opportunities. Anesthesiology. 2017;127(4):730. doi:10.1097/ALN.0000000000001795
- Thomsen JL, Nielsen C V, Palmqvist DF, Gätke MR. Premature awakening and underuse of neuromuscular monitoring in a registry of patients with butyrylcholinesterase deficiency. Br J Anaesth. 2015;115 Suppl:i89-i94. doi:10.1093/bja/aev103
- Phillips S, Stewart PA, Bilgin AB. A survey of the management of neuromuscular blockade monitoring in Australia and New Zealand. Anaesth Intensive Care. 2013;41(3):374-379. doi:10.1177/0310057X1304100316
- Ballard N, Robley L, Barrett D, Fraser D, Mendoza I. Patients’ recollections of therapeutic paralysis in the intensive care unit. Am J Crit care an Off Publ Am Assoc Crit Nurses. 2006;15(1):86-94; quiz 95.
- Workum JD, Janssen SH V, Touw HRW. Considerations in Neuromuscular Blockade in the ICU: A Case Report and Review of the Literature. Waxman KS, ed. Case Reports Crit Care. 2020;2020:8780979. doi:10.1155/2020/8780979
- Wang W, Xu C, Ma X, Zhang X, Xie P. Intensive Care Unit-Acquired Weakness: A Review of Recent Progress With a Look Toward the Future. Front Med. 2020;7:559789. doi:10.3389/fmed.2020.559789
- Eriksson LI. Evidence-based practice and neuromuscular monitoring: it’s time for routine quantitative assessment. Anesthesiology. 2003;98(5):1037-1039. doi:10.1097/00000542-200305000-00002
- Laitinen M, Sarkela M. Understanding the Myths Surrounding Neuromusclar Blockade-Reversal Agents.
- Iwasaki H, Nemes R, Brull SJ, Renew JR. Quantitative Neuromuscular Monitoring: Current Devices, NewTechnological Advances, and Use in Clinical Practice. Curr Anesthesiol Rep. 2018;8(2):134-144. doi:10.1007/ s40140-018-0261-x
- Todd MM, Hindman BJ, King BJ. The implementation of quantitative electromyographic neuromuscular monitoring in an academic anesthesia department. Anesth Analg. 2014;119(2):323-331. doi:10.1213/ ANE.0000000000000261
- Edwards L-A, Ly N, Shinefeld J, Morewood G. Universal quantitative neuromuscular blockade monitoring at an academic medical center—A multimodal analysis of the potential impact on clinical outcomes and total cost of care. Perioper Care Oper Room Manag. 2021;24:100184. doi:https://doi.org/10.1016/j.pcorm.2021.100184
- De Jong A, Molinari N, Pouzeratte Y, et al. Difficult intubation in obese patients: incidence, risk factors, and complications in the operating theatre and in intensive care units. Br J Anaesth. 2015;114(2):297-306. doi:10.1093/bja/aeu373
- Papazian L, Forel JM, Gacouin A, et al; ACURASYS Study Investigators: Neuromuscular blockers in early acute respiratory distress syndrome. N Engl J Med 2010; 363:1107–1116
- Arroliga AC, Thompson BT, Ancukiewicz M, et al; Acute Respiratory Distress Syndrome Network: Use of sedatives, opioids, and neuromuscular blocking agents in patients with acute lung injury and acute respiratory distress syndrome. Crit Care Med 2008; 36:1083–1088
- Forel JM, Roch A, Marin V, et al: Neuromuscular blocking agents decrease inflammatory response in patients presenting with acute respiratory distress syndrome. Crit Care Med 2006; 34:2749–2757
- Arroliga A, Frutos-Vivar F, Hall J, et al; International Mechanical Ventilation Study Group: Use of sedatives and neuromuscular blockers in a cohort of patients receiving mechanical ventilation. Chest 2005; 128:496–506
- Gainnier M, Roch A, Forel JM, et al: Effect of neuromuscular blocking agents on gas exchange in patients presenting with acute respiratory distress syndrome. Crit Care Med 2004; 32:113–119
- Lagneau F, D’honneur G, Plaud B, et al: A comparison of two depths of prolonged neuromuscular blockade induced by cisatracurium in mechanically ventilated critically ill patients. Intensive Care Med 2002; 28:1735–1741
- Conti G, Vilardi V, Rocco M, et al: Paralysis has no effect on chest wall and respiratory system mechanics of mechanically ventilated, sedated patients. Intensive Care Med 1995; 21:808–812
- Bishop MJ: Hemodynamic and gas exchange effects of pancuronium bromide in sedated patients with respiratory failure. Anesthesiology 1984; 60:369–371
- Kesler SM, Sprenkle MD, David WS, et al: Severe weakness complicating status asthmaticus despite minimal duration of neuromuscular paralysis. Intensive Care Med 2009; 35:157–160
- Adnet F, Dhissi G, Borron SW, et al: Complication profiles of adult asthmatics requiring paralysis during mechanical ventilation. Intensive Care Med 2001; 27:1729–1736
- Behbehani NA, Al-Mane F, D’yachkova Y, et al: Myopathy following mechanical ventilation for severe acute asthma: The role of muscle relaxants and corticosteroids. Chest 1999; 115:1627–1631
- Leatherman JW, Fluegel WL, David WS, et al: Muscle weakness in mechanically ventilated patients with severe asthma. Am J Respir Crit Care Med 1996; 153:1686–1690
- Griffin D, Fairman N, Coursin D, et al: Acute myopathy during treatment of status asthmaticus with corticosteroids and steroidal muscle relaxants. Chest 1992; 102:510–514
- Cheatham ML, Safcsak K: Is the evolving management of intraabdominal hypertension and abdominal compartment syndrome improving survival? Crit Care Med 2010; 38:402–407
- Davies J, Aghahoseini A, Crawford J, et al: To close or not to close? Treatment of abdominal compartment syndrome by neuromuscular blockade without laparostomy. Ann R Coll Surg Engl 2010; 92:W8–W9
- De Waele J, Delaet I, Hoste E, et al. The effect of neuromuscular blockers on intraabdominal pressure. Crit Care Med 2006; 34:A70
- Schramm WM, Papousek A, Michalek-Sauberer A, et al: The cerebral and cardiovascular effects of cisatracurium and atracurium in neurosurgical patients. Anesth Analg 1998; 86:123–127
- Schramm WM, Jesenko R, Bartunek A, et al: Effects of cisatracurium on cerebral and cardiovascular hemodynamics in patients with severe brain injury. Acta Anaesthesiol Scand 1997; 41: 1319–1323
- Prielipp RC, Robinson JC, Wilson JA, et al: Dose response, recovery, and cost of doxacurium as a continuous infusion in neurosurgical intensive care unit patients. Crit Care Med 1997; 25: 1236–1241
- Hsiang JK, Chesnut RM, Crisp CB, et al: Early, routine paralysis for intracranial pressure control in severe head injury: Is it necessary? Crit Care Med 1994; 22:1471–1476
- Renew, J.R., Ratzlaff, R., Hernandez-Torres, V. et al. Neuromuscular blockade management in the critically Ill patient. j intensive care 8, 37 (2020).
- Naguib M, Brull SJ, Kopman AF, Hunter JM, Fulesdi B, Arkes HR, et al. Consensus statement on perioperative use of neuromuscular monitoring. Anesth Analg. 2018;127(1):71–80.
Not all products or features are available in all markets. Full product technical specification is available upon request. Contact a HealthCare Representative for more information. Please visit www. gehealthcare.com/promotional-locations.
Data subject to change.
© 2023 HealthCare.
GE is a trademark of General Electric Company used under trademark license.
Reproduction in any form is forbidden without prior written permission from GE HealthCare. Nothing in this material should be used to diagnose or treat any disease or condition. Readers must consult a healthcare professional.
JB25849XX 12/2023
Dr. Luis Tollinche
Dr. Luis Tollinche is an anesthesiologist in New York, NY and is affiliated with Memorial Sloan-Kettering Cancer Center. He received his medical degree from Case Western Reserve University School of Medicine and has been in practice 17 years. He specializes in pediatric anesthesiology.
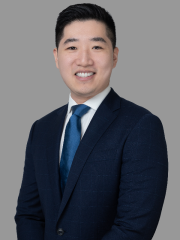
Kevin Y. Zhu
Kevin Y. Zhu is a fourth year medical student at Case Western Reserve University School of Medicine in Cleveland, OH. He grew up in Kalamazoo, MI and attended college at the University of Michigan. In college, he was inducted into Phi Beta Kappa and graduated summa cum laude with a bachelor of science and a double major in Biomolecular Studies and Asian Studies. He has several peer-reviewed publications and has made many national presentations in the fields of anesthesiology and orthopaedic surgery.