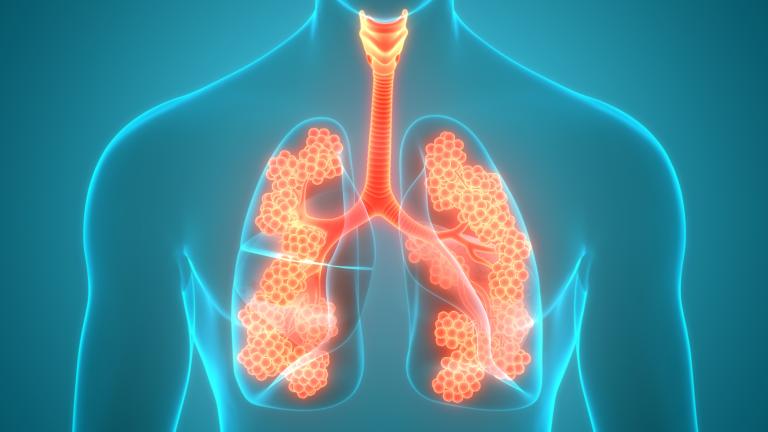
Introduction
Prior to surgery, a patient transitioning from a standing to a supine position along with the induction of general anesthesia (GA) can significantly reduce lung function due to progressive atelectasis. Shifts in the distribution of abdominal pressure, chest wall compliance, and neuromuscular blockade all produce additive and deleterious reductions in the lung’s resting, end-expiratory volume, known as functional residual capacity (FRC). As a result of these changes, the lung has a smaller volume, which functions on a flatter, less compliant portion of its pressure versus volume curve. As shown in Figure 1, to maintain the same incremental tidal volume (see normal-lung zone volume changes in Figure. 1), the lung then requires larger pressures. This shift downwards on the lung compliance curve is magnified by factors such as obesity, Trendelenburg position, and abdominal cavity insufflation.
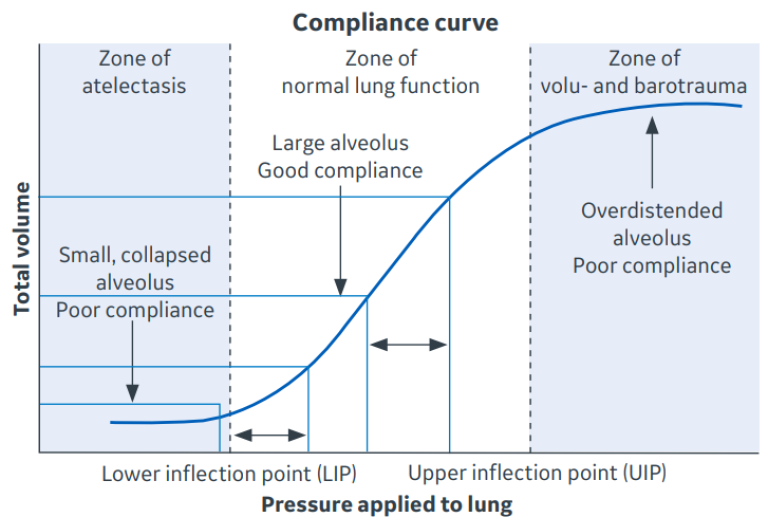
Figure 1. Lung Compliance Curve. Plotting the pressure versus volume of the lung during incremental inflation yields the compliance curve. The sigmoid shape shows that lung compliance is best in the middle zone of a normal functioning lung. Atelectasis and overdistention are associated with a flattening of the curve, indicating greater pressure is required to produce the same tidal volume. Two equal tidal volumes are shown. The tidal volume delivered in the zone of good compliance requires less pressure than the same volume delivered in the zone of atelectasis. Ventilating the lung when compliance is reduced can result in injury.
From: Walton, J. J. (2015). Advanced ventilation management. Surgery (Oxford), 33(10), 485-490.
Atelectasis during anesthesia
90% of patients undergoing GA develop hypoxemia due to progressive atelectasis. Early studies found that the use of a tidal volume (VT) of 12 – 15 mL/kg body weight was effective in reducing atelectasis and restoring compliance for normal pulmonary gas exchange in the operating room (OR).1 More recently, the adverse effects of high VT ventilation have been recognized both in patients with acute respiratory distress syndrome (ARDS) and those with healthy lungs undergoing anesthesia and surgery.2 As a result, current recommendation for lung protective ventilation in the OR include the use of lower VT ventilation, defined as 6 – 8 mL/kg predicted body weight (PBW).
While reduced VT ventilation reduces the risk of alveolar injury owing to overinflation, i.e. volutrauma or barotrauma, there is still a risk of lung injury from the cyclical collapse and reopening of underinflated lung units, a process referred to as atelectrauma. The concepts of lung stress and volumetric strain are useful in understanding the roles of atelectasis, VT, and FRC in mediating lung injury and in preventing atelectrauma.
Mechanical effects of atelectasis
Stress and strain are terms used in materials engineering when qualifying the dynamic forces acting upon a material. These concepts can be used to describe the forces acting upon the lung during positive pressure mechanical ventilation. Stress is defined as force per area; when a given VT is delivered to the lung, the stress associated with that VT will increase as the area of the lung is reduced, such as occurs with atelectasis. Strain is defined as change in length of a material divided by its original length, for example, when a spring is stretched beyond its resting length. Volumetric strain refers to volume rather than length and is useful in describing the forces acting on an alveolus during a mechanical breath. Volumetric strain of the entire lung can therefore be expressed as lung volume change (VT) divided by its original, end-expiratory volume (Figure 2). End-expiratory volume (EELV) is the same as FRC during positive pressure ventilation. The reduction in FRC (and EELV) seen with GA and amplified by obesity, positioning, and abdominal insufflation in the OR results in increased volumetric strain during mechanical ventilation.3 Excessive strain causes alveolar damage which results in an increased systemic inflammatory response and contributes to postoperative pulmonary complications. When FRC becomes significantly reduced, even low VT ventilation can potentially become injurious. For this reason, effective lung protection requires not just the use of low VT ventilation, but also restoration of normal FRC to reduce lung stress and strain.
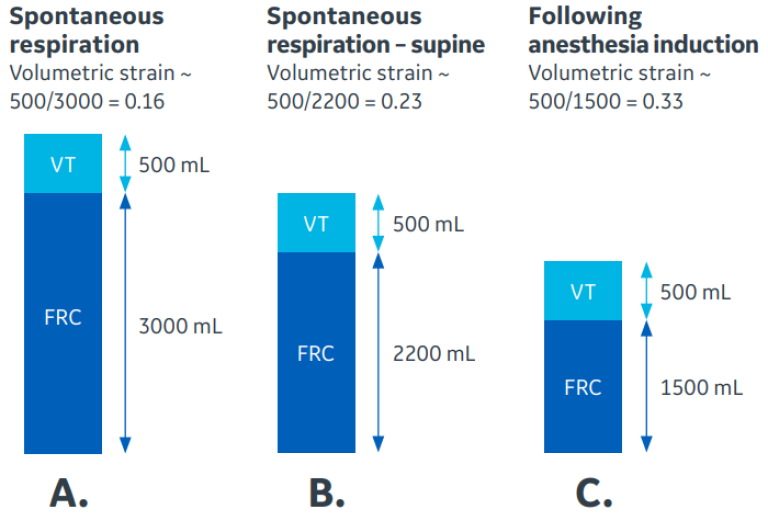
Figure 2. Effect of reduced functional residual capacity (FRC) on lung strain. Panel A shows normal volumetric strain of 16% during spontaneous respiration. Reduction of FRC due to supine positioning increases strain to 23% (Panel B). Induction of anesthesia further reduces FRC and increases strain to 33% (Panel C). Restoration of FRC with ARMs and PEEP can reduce lung volumetric strain.
Gas exchange impairment due to atelectasis
Collapsed alveoli result in shunting of pulmonary blood flow through the lungs. These lung units are unable to contribute to ventilation and result in impaired CO2 removal and poorly oxygenated blood returning directly to the systemic circulation, creating hypercarbia and hypoxemia that is refractory to increased fraction of inspired oxygen (FiO2). As more atelectasis develops, the amount of blood being shunted directly to the systemic circulation increases, further worsening hypercarbia and hypoxemia.4
Driving pressure
Driving pressure is the pressure delivered to the lungs during a mechanical breath. It is the difference between the plateau inspiratory pressure and the end-expiratory pressure (Figure 3). It can be measured using a volume-controlled breath with an inspiratory pause. During the pause, there is zero flow, and measured plateau pressure (Pplat) will correlate more accurately with alveolar pressure than peak airway pressure. Pplat can be estimated by using peak airway pressure during pressure modes of controlled mechanical ventilation when the end-expiratory flow is back at zero, which is visible on the flow waveform of the anesthesia machine display. Driving pressure can be monitored continuously during anesthesia and provides a target for lung protection. As the lungs become less compliant due to volume loss, higher pressures are required to produce the same tidal volume. Lung stress and volumetric strain are increased as a result. Reducing VT can help, but at the expense of further hypoxemia and/or hypercarbia. Increased driving pressures are associated with higher mortality rates in patients with ARDS.5 Levels greater than 14 – 15 cmH2O have been shown to be significantly associated with the development of postoperative complications after GA.6 Even small changes in driving pressure can have significant impact on clinical outcomes. A meta-analysis of 17 randomized controlled trials of lung protective ventilation showed an independent association between postoperative pulmonary complications and increased driving pressure or increases in driving pressure associated with changes in positive end-expiratory pressure (PEEP).7 Each 1 cmH2O increase in driving pressure increased the odds of developing a postoperative pulmonary complication by 16%.
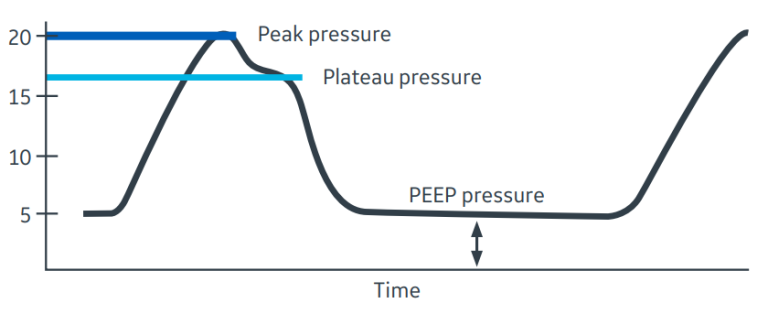
Figure 3. Driving Pressure. Driving Pressure is determined by the difference between plateau pressure (light blue line) and end-expiratory pressure. In this example, a single volume-controlled breath is shown. The plateau pressure of 16 cmH2O can be determined during an end-inspiratory pause. PEEP is 5 cmH20, giving a driving pressure of 11 cmH2O. Note that the peak inspiratory pressure, which is influenced by gas flow rate and airway resistance, overestimates driving pressure.
Opening pressure and ARMs
A collapsed alveolus can be returned to its normal functioning state with the application of sufficient positive pressure. The opening pressure of an alveolus or terminal airway is the specific amount of positive pressure that results in their reopening. The amount of pressure required to recruit a collapsed unit varies from alveolus to alveolus. When appropriate levels of positive pressure are applied and opening pressure is achieved and briefly maintained, alveolar recruitment is obtained. During GA, most collapsed alveoli can be opened with an alveolar recruitment maneuver (ARM) of 40 cmH2O for 7 – 10 seconds.8 Higher levels may be necessary with higher BMI individuals.9
Recruitment maneuvers can be accomplished in several ways. During anesthesia, the adjustable pressure-limiting (APL) valve can be switched to bag mode and an ARM can be delivered manually. However, this method is NOT recommended for a few reasons. First, switching the APL from bag to ventilator can result in a transient release of pressure causing de-recruitment of the just opened alveoli. More importantly, the “bag squeezing” ARM has been associated with increased rates of postoperative pulmonary complications.9 Methods that use step-wise changes in ventilator settings are preferred. One can increase mechanically delivered volume and/or pressure every 3 – 6 breaths until target opening pressure is achieved. After delivering several breaths at the target pressure, baseline low VT ventilation can be resumed.
The safety of step-wise recruitment of surgical patients has been shown in multiple studies.10 The most common complication of an ARM is the transient need for vasopressor support. Rates of significant hemodynamic effects and barotrauma do not appear increased when ARMs are used appropriately in the OR.
The “open lung”
ARMs alone do not maintain inflation of alveoli that are prone to collapse. Once a collapsed alveolus is re-opened with an ARM, sufficient PEEP is required to maintain it in the “open lung” state.11 The amount of PEEP needed to maintain the open lung once alveoli are restored to their functional state varies from individual to individual and can also change during a surgical procedure due to dynamic changes in abdominal pressure and chest wall compliance associated with changes in positioning, retractor placement, and abdominal insufflation. One common method of determining the correct level of PEEP is to perform an ARM and then perform a gradual downward PEEP titration. The PEEP that produces the lowest driving pressure during volume-controlled ventilation (or the best tidal volume for a given pressure in pressure-controlled ventilation) corresponds to the best compliance of the lung and balances tidal volume between over- and under-inflation of the lung. A second ARM is then performed, and PEEP set at 1 – 2 cmH2O above that identified level (Figure 4). It has been shown that a median PEEP of 8 – 10 cmH2O is needed to minimize driving pressure during abdominal surgery in normal body mass index (BMI) individuals.12
Individualized PEEP, as determined by minimizing driving pressure during open abdominal procedures, has been shown to decrease intraoperative atelectasis, improve oxygenation during and after surgery, and significantly reduce postoperative pulmonary complications.13 Studies of patients with normal BMIs undergoing laparoscopic procedures have found that optimal PEEP is 10 – 14 cmH2O, and 2 – 4 cmH2O more is needed during Trendelenburg positioning and abdominal insufflation.14 Higher levels of individualized PEEP, up to 26 cmH2O, have been found to be needed to maintain FRC, improve oxygenation, and lower driving pressure in higher BMI individuals undergoing open abdominal surgery.15 Lung recruitment that restores lost FRC and reduces the deleterious effects of lost FRC requires the use of both ARMs and PEEP.
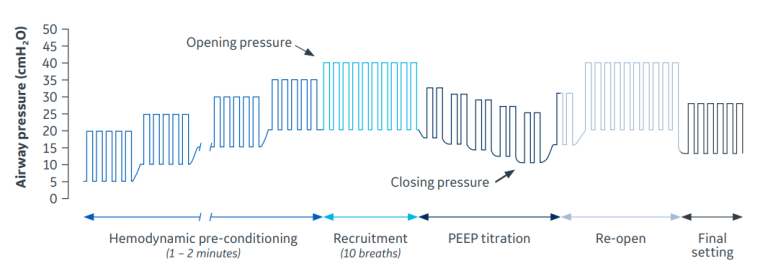
Figure 4. Alveolar Recruitment Maneuvers Strategy (ARMS). The ARMS is performed in pressure control ventilation with a driving pressure (plateau – PEEP) of 15 cmH2O. Each rectangle represents a tidal volume. PEEP is increased in increments of 5 cmH2O during the hemodynamic pre-conditioning phase. Then PEEP and driving pressure are increasing to 20 cmH2O to reach the opening pressure of the lungs. After 10 breaths a complete open lung state is achieved. The PEEP titration trial starts reducing the driving pressure to 15 cmH2O, and then reducing PEEP in increments of 2 cmH2O, from 20 cmH2O until the closing pressure is found. When subsequent recruitment maneuvers are performed, the patient is then ventilated with a protective setting (low VT), but at the established Open Lung-PEEP.
From: Tusman, Gerardo, and Javier F. Belda. “Treatment of anesthesia-induced lung collapse with lung recruitment maneuvers.” Current anaesthesia & critical care 21, no. 5-6 (2010): 244-249.
Expert panel consensus
A panel of international experts in intraoperative mechanical ventilation recently published consensus statements regarding ARMs.16 33 studies of ARMs in the OR were selected using the GRADE method and discussed under Delphi guidelines. The panel concluded that:
- ARMs should be performed after a disconnection from the circuit and whenever the patient’s hemoglobin saturation is consistently ≤94%.
- One should evaluate change in respiratory system compliance and driving pressure after an ARM and repeat the ARM with a longer inspiratory hold or higher pressure if recruitment is assessed as ineffective.
- ARMs should be performed using the lowest effective peak inspiratory pressure and shortest effective time or fewest number of breaths.
Conclusion
Restoring lung volume has become increasingly recognized as the basis for safe administration of mechanical ventilation in the operating room (OR). Healthy lungs can be exposed to significant stress and strain during routine mechanical ventilation in the OR due to reductions in FRC. Obesity, head down positioning, and abdominal insufflation are among the factors that can further compromise end-expiratory lung volume. The restoration of FRC or EELV can be safely accomplished with individualized application of positive end-expiratory pressure (PEEP) coupled with recruitment maneuvers (RM). Collapsed alveoli and terminal airways can be safely returned to their normally inflated “open lung” state, which reduces ventilator driving pressure. Furthermore, there is growing evidence that intraoperative improvement in respiratory function resulting from the open lung approach may reduce postoperative pulmonary complications. Extending open lung management into the immediate postoperative setting may also provide outcome benefits.17
References
- Bendixen, H. H., Whyte, H., & Laver, M. B. (1963). Impaired oxygenation in surgical patients during general anesthesia with controlled ventilation: a concept of atelectasis. New England Journal of Medicine, 269(19), 991-996.
- Futier, E., Constantin, J. M., Paugam-Burtz, C., Pascal, J., Eurin, M., Neuschwander, A., ... & Jaber, S. (2013). A trial of intraoperative low-tidal-volume ventilation in abdominal surgery. New England Journal of Medicine, 369(5), 428-437.
- Brunner, J. X., & Wysocki, M. (2012). Is there an optimal breath pattern to minimize stress and strain during mechanical ventilation? In Applied Physiology in Intensive Care Medicine 1 (pp. 25-29). Springer, Berlin, Heidelberg.
- Hedenstierna, G., & Rothen, H. U. (2000). Atelectasis formation during anesthesia: causes and measures to prevent it. Journal of clinical monitoring and computing, 16(5), 329-335.
- Guérin, C., Papazian, L., Reignier, J., Ayzac, L., Loundou, A., & Forel, J. M. (2016). Effect of driving pressure on mortality in ARDS patients during lung protective mechanical ventilation in two randomized controlled trials. Critical Care, 20(1), 1-9.
- Ladha, K., Melo, M. F. V., McLean, D. J., Wanderer, J. P., Grabitz, S. D., Kurth, T., & Eikermann, M. (2015). Intraoperative protective mechanical ventilation and risk of postoperative respiratory complications: hospital-based registry study. Bmj, 351.
- Neto, A. S., Hemmes, S. N., Barbas, C. S., Beiderlinden, M., FernandezBustamante, A., Futier, E., ... & PROVE Network Investigators. (2016). Association between driving pressure and development of postoperative pulmonary complications in patients undergoing mechanical ventilation for general anaesthesia: a meta-analysis of individual patient data. The Lancet Respiratory Medicine, 4(4), 272-280.
- H. U. Rothen, P. Neumann, J. E. Berglund, J. Valtysson, A. Magnusson and G. Hedenstierna. Dynamics of re-expansion of atelectasis during general anaesthesia. British Journal of Anaesthesia 82 (4): 551–6 (1999).
- Ball, L., Hemmes, S. N. T., Neto, A. S., Bluth, T., Canet, J., Hiesmayr, M., ... & Pelosi, P. (2018). Intraoperative ventilation settings and their associations with postoperative pulmonary complications in obese patients. British Journal of Anaesthesia, 121(4), 899-908.
- Tusman, G., & Belda, J. F. (2010). Treatment of anesthesia-induced lung collapse with lung recruitment maneuvers. Current anaesthesia & critical care, 21(5-6), 244-249.
- Lachmann, B. (1992). Open up the lung and keep the lung open. Intensive care medicine, 18(6), 319-321.
- Ferrando, C., Suarez-Sipmann, F., Tusman, G., León, I., Romero, E., Gracia, E., ... & Belda, F. J. (2017). Open lung approach versus standard protective strategies: Effects on driving pressure and ventilatory efficiency during anesthesia-A pilot, randomized controlled trial. PloS one, 12(5), e0177399.
- Zhang, C., Xu, F., Li, W., Tong, X., Xia, R., Wang, W., ... & Shi, X. (2021). Driving Pressure–Guided Individualized Positive End-Expiratory Pressure in Abdominal Surgery: A Randomized Controlled Trial. Anesthesia & Analgesia, 133(5), 1197-1205.
- Pereira, S. M., Tucci, M. R., Morais, C. C., Simões, C. M., Tonelotto, B. F., Pompeo, M. S., ... & Amato, M. B. (2018). Individual positive end-expiratory pressure settings optimize intraoperative mechanical ventilation and reduce postoperative atelectasis. Anesthesiology, 129(6), 1070-1081.
- Simon, P., Girrbach, F., Petroff, D., Schliewe, N., Hempel, G., Lange, M., ... & Wrigge, H. (2021). Individualized versus Fixed Positive End-expiratory Pressure for Intraoperative Mechanical Ventilation in Obese Patients: A Secondary Analysis. Anesthesiology, 134(6), 887-900.
- Young, C. C., Harris, E. M., Vacchiano, C., Bodnar, S., Bukowy, B., Elliott, R. R. D., ... & Sprung, J. (2019). Lung-protective ventilation for the surgical patient: international expert panel-based consensus recommendations. British Journal of Anaesthesia, 123(6), 898-913.
- Futier, E., Constantin, J. M., Pelosi, P., Chanques, G., Massone, A., Petit, A., ... & Jaber, S. (2011). Noninvasive ventilation and alveolar recruitment maneuver improve respiratory function during and after intubation of morbidly obese patients: a randomized controlled study. The Journal of the American Society of Anesthesiologists, 114(6), 1354-1363.
© 2022 General Electric Company.
GE and the GE Monogram are trademarks of General Electric Company. GE Healthcare, a division of GE. GE Medical Systems, Inc., doing business as GE Healthcare. Reproduction in any form is forbidden without prior written permission from GE. The opinions, beliefs and viewpoints expressed in this article are solely those of the author and do not necessarily reflect the opinions, beliefs and viewpoints of GE Healthcare. The author is a paid consultant for GE Healthcare and was compensated for creation of this article. Use of this material is limited to agents and employees of GE Healthcare or other parties expressly licensed by GE. Unlicensed use is strictly prohibited. This material may contain clinical concepts and definitions. No diagnostic statements are inferred or included in this material. All clinical diagnosis should be made by a trained healthcare professional.
August 2022
JB20375XX
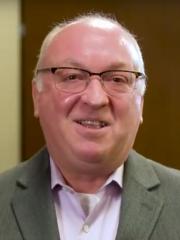