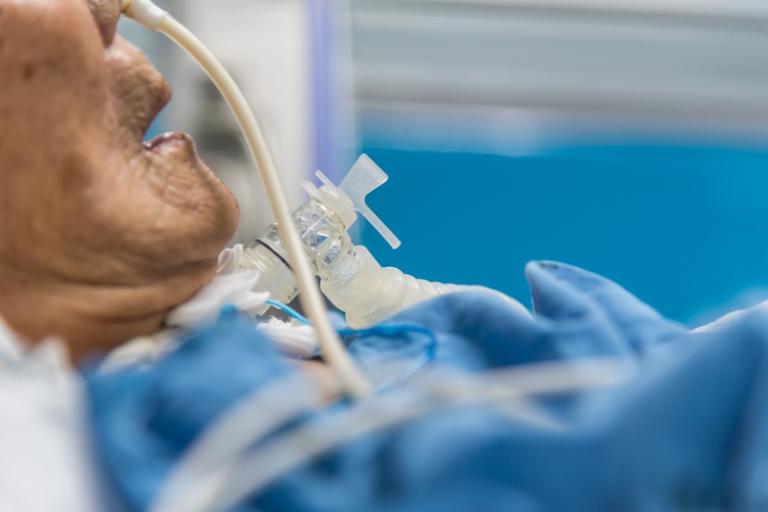
While mechanical ventilation can be a life-saving intervention, it also carries a host of risks, including lung injury, which can lead to pulmonary edema, worsen hypoxia and barotrauma and perpetuate the need for the ventilation itself, increasing the chance of multi-system organ failure, as well as morbidity and mortality1. Because of these dangers, adopting ventilator strategies that mitigate ventilator-induced lung injury or VILI is an elemental objective in ventilatory control.
In this article, we’ll discuss the dangers of VILI and the mechanisms behind its development. We’ll also delve into the precautions that should be implemented to mitigate its risks in order to provide safe and adequate ventilation.
Understanding VILI
Mechanical ventilation is required to reinforce respiratory functions in diverse clinical conditions, ranging from diseased to healthy lungs. However, recent, research has indicated that such mechanical ventilation could perpetuate chronic and acute destruction to pulmonary structures through what is known as VILI2.
VILI delineates an acute lung injury, which typically affects parenchyma and airways2. The key features of VILI include lung strain and alveolar over-distention, which reflect the presence of increased transpulmonary pressure, or the difference between pleural and airway pressure, at the culmination of an inspiration.
The Mechanisms Behind VILI
The mechanisms which may induce VILI are complex, involving both biochemical and biophysical factors. Fundamentally, three biophysical mechanisms underlie VILI pathogenesis3.
These include3:
• Barotrauma
• Atelectrauma
• Volutrauma
While volutrauma defines an injury brought about by about by alveolar over-distention, barotrauma occurs as a result of excessive transpulmonary pressure3. In turn, atelectrauma delineates injury that occurs due to shear stress caused by the repeated opening and closing of the alveoli.3
Additionally, biotrauma is also a factor in VILI, involving the release of various pro-inflammatory mediators due to cellular response that occur in response to the three biophysical injuries.3
At its core, VILI encompasses alveolar instability and lung inhomogeneity, popularly known as uneven lung aeration. These interact with each other to cause VILI development. More specifically, inhomogeneity places a patient’s lungs at increased alveolar over-distention risk, which predisposes them to volutrauma and barotrauma3. Worse still, alveolar instability encourages atelectrauma development.
Primarily, researchers believed VILI to be caused by excessive airway pressure, commonly known as barotrauma. However, recent findings assert that the major cause of VILI to be excessive cyclic volume alterations, or volutrauma, which occur during ventilation. Based on this over-distention injury understanding, researchers have found that inhomogeneously aerated lung regions secondary to atelectasis lack a protective mechanism provided by the alveoli inflation3. Typically, when alveoli get adequate inflation, their resultant structure safeguards the lungs from injury through uniform stress distribution.
Another important VILI mechanism involves alveolar interdependence. Essentially, neighboring alveoli are mechanically interdependent, sharing the inter-alveolar septum. As such, the deformation of any alveolus, whether due to liquid-filling or collapse, leads to deformation of adjacent alveolus, resulting in greater regional tensile forces4. This type of VILI caused by heterogeneous strain and stress distribution within the distinct alveoli, as well as across the neighboring alveolus, is known as stress raisers or stress concentration, alternatively referred to as lung inhomogeneity.4
Precautions to Minimize VILI Risk
Lung protective ventilation (LPV) is created to safeguard vascular endothelial and pulmonary epithelial cells against VILI mechanisms. However, this measure has a downside, since it fails to improve oxygenation. The LPV parameters have been designed primarily to maintain the stability and homogenous ventilation, coupled with including individualized lung volume (VT) parameters, inspiratory time and post end expiratory pressure (PEEP)3.
This makes additional factors vital in risk reduction for VILI, including re-establishing end-expiratory lung volume (EELV) after anesthesia via alveolar recruitment maneuvers and reducing the inspired oxygen’s fraction.3 Further research in support of LPV strategies like higher PEEP and low lung volume, indicates that they minimize repetitive closure and opening of alveolar units and lung collapse with reduced inflammatory response2.
Conversely, recent experiential evidence about permissive atelectasis to mitigate VILI asserts that low PEEP integrated with low lung volume, transpulmonary pressure and plateau pressure reduce lung injury. These findings challenged prevalent beliefs regarding atelectasis being harmful to protective ventilation2. However, this only works if lungs are at rest.
More specifically, this preventive strategy entails various elements. The first involves keeping PEEP at minimal levels to ensure enough gas exchange. This allows a certain permissive hypoxemia level to guarantee oxygen saturation above 88%2. The low PEEP level linked to low lung volume manages to ventilate aerated lungs discriminatively, while simultaneously reducing the detrimental effects likely to affect collapsed peripheral airways and alveoli.
The second involves setting the respiratory rate in a manner that ensures the arterial pH (pHa) stays within the physiological ranges. This permissive atelectasis strategy integrates protective lung effects and mitigates the probable hemodynamic impairment.2
In addition to lung unit recruitment, which increases shrinking (baby) lungs (BL) coupled with helping diffuse strain and stress across the aerated network, a primary benefit linked to moderate and low PEEP application in Acute Respiratory Disease (ARDs) management involves providing counter-pressure. The counter-pressure, in turn, trans-locates and redistributes alveolar edema from alveoli to intestinal matrices5. As a result, PEEP brakes, prevents or interrupts tidal trans-airway propagation of mediator-laden fluid and protein. On the other hand, unnecessarily high PEEP levels typically lead to negative consequences like increased ventilating power, augmented stress on open BL units and the hemodynamic alteration of systemic crucial organs, especially when the patient is in a supine position5.
On the other hand, the implementation of prone positioning may evenly disperse the regional transpulmonary forces. This makes proning a key intervention to enhance ventilation and perfusion (V/Q) matching as well as avoid the regional tidal strains application past the VILI threshold5.
Conclusion
Lung injury is an adverse complication caused by mechanical ventilation, which can be life-threatening. Mechanisms that may perpetuate VILI include volutrauma, atelectrauma and barotrauma and may result in dangerous sequelae, including pulmonary edema and hypoxia and may worsen morbidity mortality1.
Inherently, it becomes imperative to develop measures to mitigate the risks associated with VILI in order to improve patient care and safety. Such measures include lung protective ventilation, permissive atelectasis and lung unit recruitment. Clinical utilization of reduced tidal volume to attain minimized inspiratory strain and stress, integrated with increased positive end-expiratory pressure (PEEP) to evade recurrent reopening and collapse of alveolar units are protective ventilatory measures that could help protect patients from VILI.
References
1: Ajith Kumar, A. K., & Anjum, F. (2022). Ventilator-Induced Lung Injury (VILI). In StatPearls. StatPearls Publishing.
2: Pelosi, P., Rocco, P. R., & Gama de Abreu, M. (2018). Close down the lungs and keep them resting to minimize ventilator-induced lung injury. Critical Care, 22(1). https://doi.org/10.1186/s13054-018-1991-3
3: Tsumura, H. (2021). Review of the Mechanisms of Ventilator Induced Lung Injury and the Principles of Intraoperative Lung Protective Ventilation. AANA journal, 89(3).
4: Madahar, P., & Beitler, J. R. (2020). Emerging concepts in ventilation-induced lung injury. F1000Research, 9, 222. https://doi.org/10.12688/f1000research.20576.
5: Marini, J. J., & Gattinoni, L. (2020). Time course of evolving ventilator-induced lung injury: The “Shrinking baby lung”. Critical Care Medicine, 48(8), 1203-1209. https://doi.org/10.1097/ccm.0000000000004416
© 2023 GE HealthCare
GE is a trademark of General Electric Company used under trademark license. Reproduction in any form is forbidden without prior written permission from GE HealthCare. Nothing in this material should be used to diagnose or treat any disease or condition. Readers must consult a healthcare professional.